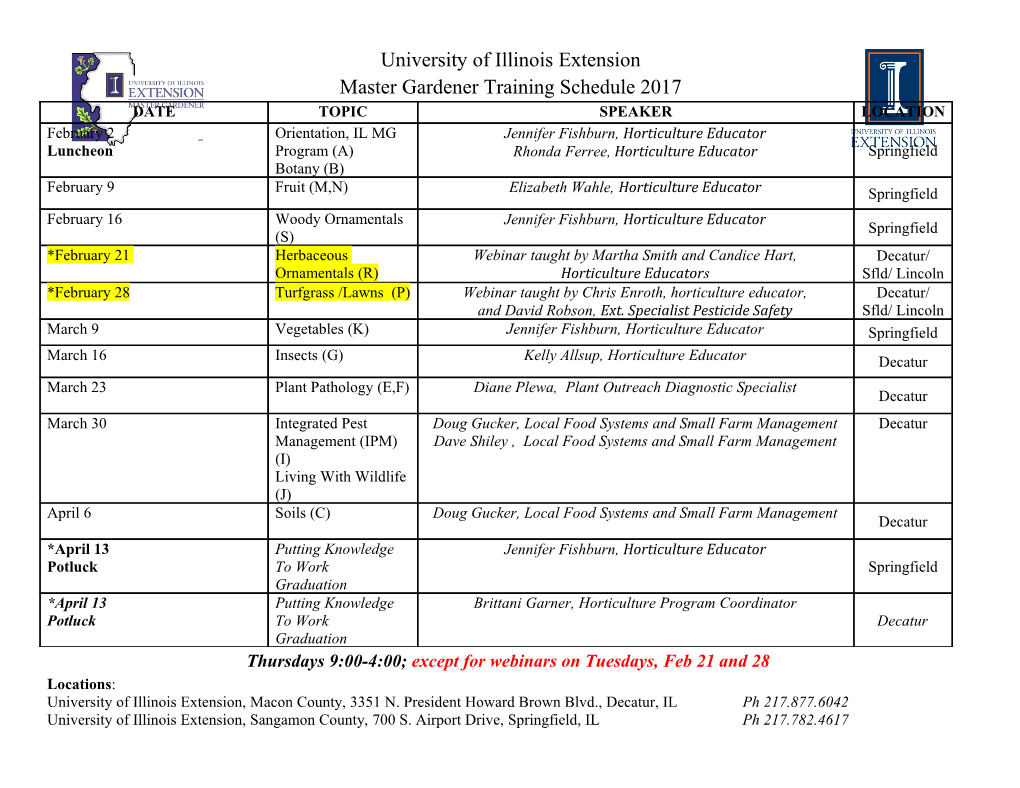
University of Rhode Island DigitalCommons@URI Graduate School of Oceanography Faculty Graduate School of Oceanography Publications 2018 Radiolytic H2 Production in Martian Environments Mary Dzaugis university of rhode island, [email protected] Arthur J. Spivack University of Rhode Island, [email protected] See next page for additional authors Creative Commons License This work is licensed under a Creative Commons Attribution 4.0 License. Follow this and additional works at: https://digitalcommons.uri.edu/gsofacpubs Citation/Publisher Attribution Dzaugis, M., Spivack, A.J., D'Hondt, S. Radiolytic H2 production in martian environments (2018) Astrobiology, 18(9), pp. 1137-1146. DOI: 10.1089/ast.2017.1654 This Article is brought to you for free and open access by the Graduate School of Oceanography at DigitalCommons@URI. It has been accepted for inclusion in Graduate School of Oceanography Faculty Publications by an authorized administrator of DigitalCommons@URI. For more information, please contact [email protected]. Authors Mary Dzaugis, Arthur J. Spivack, and Steven D’Hondt This article is available at DigitalCommons@URI: https://digitalcommons.uri.edu/gsofacpubs/429 ASTROBIOLOGY Volume 18, Number 9, 2018 Mary Ann Liebert, Inc. DOI: 10.1089/ast.2017.1654 Radiolytic H2 Production in Martian Environments Mary Dzaugis, Arthur J. Spivack, and Steven D’Hondt Abstract Hydrogen, produced by water radiolysis, has been suggested to support microbial communities on Mars. We quantitatively assess the potential magnitude of radiolytic H2 production in wet martian environments (the ancient surface and the present subsurface) based on the radionuclide compositions of (1) eight proposed Mars 2020 landing sites, and (2) three sites that individually yield the highest or lowest calculated radiolytic H2 production rates on Mars. For the proposed landing sites, calculated H2 production rates vary by a factor of *1.6, while the three comparison sites differ by a factor of *6. Rates in wet martian sediment and micro- fractured rock are comparable with rates in terrestrial environments that harbor low concentrations of microbial life (e.g., subseafloor basalt). Calculated H2 production rates for low-porosity (<35%), fine-grained martian sediment (0.12–1.2 nM/year) are mostly higher than rates for South Pacific subseafloor basalt (*0.02–0.6 nM/ year). Production rates in martian high-porosity sediment (>35%) and microfractured (1 mm) hard rock (0.03 to <0.71 nM/year) are generally similar to rates in South Pacific basalt, while yields for larger martian fractures (1 and 10 cm) are one to two orders of magnitude lower (<0.01 nM/year). If minerals or brine that amplify radiolytic H2 production rates are present, H2 yields exceed the calculated rates. Key Words: Mars—Water radiolysis—Habitability—Hydrogen—Geochemistry—Mars 2020. Astrobiology 18, 1137–1146. 1. Introduction Previous studies suggested that radiolysis might support life on Mars (i.e.,Linet al., 2005b; Sherwood Lollar et al., he search for extraterrestrial life has prompted >20 2007; Lefticariu et al., 2010; Tu¨rke et al., 2015). However, Tsurface and orbital missions to Mars (Walter, 2015). few studies quantified radiolysis rates. To better under- Many of these missions have focused on identifying past and stand the origin of the subsurface methane flux on Mars, present aqueous environments, as water is necessary for life Onstott et al. (2006) investigated the diffusion of He, H2, as we know it. However, it is not the only requirement for life. and CH4 through the martian crust. They calculated ra- Habitable environments must also provide biologically har- diolytic production rates for the deep subsurface crust vestable energy. A variety of geochemical processes produce based on the chemical composition of martian meteorites chemical species that can be metabolized by microorganisms and a 6.6 km-thick crustal profile of estimated porosity. In for energy. Several studies have proposed that some sub- contrast to their global approach, we calculate specific surface microbial communities on Earth rely on molecular rates for a broad range of lithologies, including both water- hydrogen (H2) as their primary electron donor (i.e., Stevens saturated rock and sediment, based on compositional data and McKinley, 1995; Pedersen, 2000). In terrestrial subsur- at 11 specific sites on Mars. face environments, H2 is abiologically produced by water These 11 sites include the three currently proposed Mars radiolysis (i.e., Pedersen, 2000; Lin et al., 2005a; Blair et al., 2020 Rover landing sites (Witze, 2017), five other sites that 2007; Tu¨rke et al., 2015; Dzaugis et al., 2016), rock weath- were previously under consideration for the Mars 2020 ering (i.e., Stevens and McKinley, 1995; Pedersen, 2000), mission (Ono et al., 2016), and three sites with minimum or and serpentinization (i.e., Kelley et al., 2001, 2005). These maximum mean martian rates of radiolytic H2 production chemolithotrophic communities serve as models for life on (based on radionuclide concentrations). Our purpose of se- other planets, such as Mars (McCollom and Seewald, 2013). lecting these sites is to quantify (1) the magnitude of ra- Here we quantify the potential for radiolytic production of H2 diolytic H2 production in the wet martian past at potential in water-saturated environments, such as the ancient martian Mars 2020 landing sites, and (2) the range of H2 production surface or the present subsurface. rates in present-day martian subsurface groundwater. Graduate School of Oceanography, University of Rhode Island, Narragansett, Rhode Island. Ó Mary Dzaugis et al., 2018; Published by Mary Ann Liebert, Inc. This Open Access article is distributed under the terms of the Creative Commons License (http://creativecommons.org/licenses/by/4.0), which permits unrestricted use, distribution, and reproduction in any medium, provided the original work is properly credited. 1137 1138 DZAUGIS ET AL. Identification of past and present habitable environments eration, as all eight sites may have once harbored liquid water, is one of the main objectives of Mars Exploration missions and potentially H2-oxidizing life (Ono et al., 2016). (Mustard et al., 2013). Because oxidative H2 respiration is a Finally, we chose three additional locations, based on source of chemical energy for microbial life, estimates of their radioisotope compositions, to evaluate minimum and past H2 production rates based on the radioisotope compo- maximum radiolytic H2 production rates on the past martian sitions of proposed Mars 2020 sites are important for un- surface and in the present subsurface: Acidalia Planitia derstanding the ancient habitability of the sites. (maximum rate), the northern pole (minimum rate for an Eight sites were originally considered as the possible ice-covered region), and Promethei Terra (minimum rate for landing sites for the Mars 2020 mission (Mustard et al., a region without ice cover). The locations of the 11 sites are 2013). Many of these locations have similar geological and shown in Figure 1. topographic features, in part because the final site must be Martian surface material ranges from loose, fine-grained safe for rovers to land and travel (Mustard et al., 2013). The regolith to large pieces of fractured rock. To account for this eight candidate sites were narrowed to three based on the heterogeneity, we calculate H2 production rates using two following criteria: an ancient environment likely to have been different radiolysis models. One model is for fractured hard- habitable, accessible rocks with potential for biosignature rock aquifers (Dzaugis et al., 2015, 2016), the other is for preservation, and geological materials that may answer water-saturated fine-grained sediment (Blair et al., 2007). questions about planetary evolution (Witze, 2017). The three sites currently under consideration for the 2. Methods Mars 2020 landing site are as follows: Jezero Crater, an In this section, we describe the radiolysis models and ancient lake that has both deltaic and lacustrine deposits discuss the variables used in our calculations: fractured rock with well-defined stratigraphy (Schon et al., 2012; Witze, composition, sediment porosity, radioactivity, density, and 2017); NE Syrtis Major which was once volcanically ac- H yield per 100 eV absorbed (G-value). Lithologies of the tive, with widespread evidence of past liquid-water activity 2 11 localities are described in Table 1, and calculated H and a variety of interesting environments, including a 2 production rates are given in Table 2. banded olivine-carbonate unit and a crater-retaining cap of mafic rock (Hiesinger and Head, 2004; Ono et al., 2016); 2.1. Fractured hard-rock model and Columbia Hills, where Mars Exploration rover Spirit provided evidence of ancient hydrothermal activity and/or We previously used this model to calculate production rates hot springs (Squyres et al., 2008). in terrestrial oceanic basaltic aquifers (Dzaugis et al., 2016). In In addition to these three locations, we evaluate radiolytic H2 this study, we use parameter values appropriate for Mars. production for five other sites originally under consideration Within hard-rock aquifers, ionizing radiation produced for Mars 2020: Eberswalde Crater, Holden Crater, Nili Fossae, during the decay of radioactive elements in the rock decom- Mawrth Vallis, and SW Melas Chasma. We include these sites poses water molecules and leads to production of hydrogen as points of comparison for the three sites still under consid- molecules
Details
-
File Typepdf
-
Upload Time-
-
Content LanguagesEnglish
-
Upload UserAnonymous/Not logged-in
-
File Pages12 Page
-
File Size-