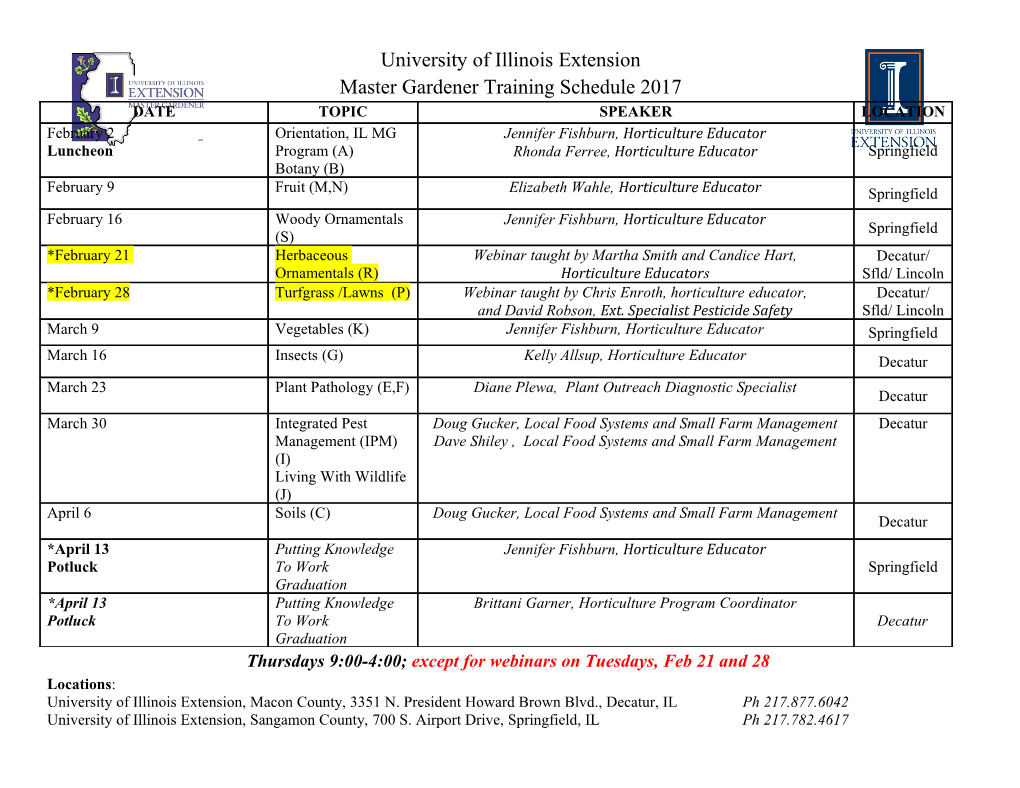
nanomaterials Article Improving the Stability of High-Voltage Lithium Cobalt Oxide with a Multifunctional Electrolyte Additive: Interfacial Analyses Xing-Qun Liao 1,2, Feng Li 2, Chang-Ming Zhang 2, Zhou-Lan Yin 1,*, Guo-Cong Liu 3,* and Jin-Gang Yu 1,3,* 1 College of Chemistry and Chemical Engineering, Central South University, Changsha 410083, China; [email protected] 2 Research Institute of Highpower International, Huizhou 516057, China; fl[email protected] (F.L.); [email protected] (C.-M.Z.) 3 School of Chemistry and Materials Engineering, Huizhou University, Huizhou 516007, China * Correspondence: [email protected] (Z.-L.Y.); [email protected] (G.-C.L.); [email protected] (J.-G.Y.); Tel./Fax: +86-731-88879616 (Z.-L.Y.); +86-731-88879616 (J.-G.Y.) Abstract: In recent years, various attempts have been made to meet the increasing demand for high energy density of lithium-ion batteries (LIBs). The increase in voltage can improve the capacity and the voltage platform performance of the electrode materials. However, as the charging voltage increases, the stabilization of the interface between the cathode material and the electrolyte will decrease, causing side reactions on both sides during the charge–discharge cycling, which seriously affects the high-temperature storage and the cycle performance of LIBs. In this study, a sulfate additive, dihydro-1,3,2-dioxathiolo[1,3,2]dioxathiole 2,2,5,5-tetraoxide (DDDT), was used as an effi- cient multifunctional electrolyte additive for high-voltage lithium cobalt oxide (LiCoO2). Nanoscale Citation: Liao, X.-Q.; Li, F.; Zhang, protective layers were formed on the surfaces of both the cathode and the anode electrodes by C.-M.; Yin, Z.-L.; Liu, G.-C.; Yu, J.-G. Improving the Stability of the electrochemical redox reactions, which greatly decreased the side reactions and improved the High-Voltage Lithium Cobalt Oxide voltage stability of the electrodes. By adding 2% (wt.%) DDDT into the electrolyte, LiCoO2 exhibited ◦ with a Multifunctional Electrolyte improved Li-storage performance at the relatively high temperature of 60 C, controlled swelling Additive: Interfacial Analyses. behavior (less than 10% for 7 days), and excellent cycling performance (capacity retention rate of Nanomaterials 2021, 11, 609. https:// 76.4% at elevated temperature even after 150 cycles). doi.org/10.3390/nano11030609 Keywords: high-voltage; lithium cobalt oxide; multifunctional electrolyte additives; interfacial stability Academic Editor: Christophe Detavernier Received: 23 December 2020 1. Introduction Accepted: 25 February 2021 Published: 28 February 2021 In the past decades, remarkable progress has been made in lithium-ion batteries (LIBs) in the field of portable devices [1,2]. In addition, LIBs can be used as efficient aerospace Publisher’s Note: MDPI stays neutral batteries for small satellites, and LIBs are becoming one of the most widely used energy with regard to jurisdictional claims in storage devices due to their relatively high working potential and high energy density [3–6]. published maps and institutional affil- However, it is well known that the energy density of cathode materials is the main factor iations. affecting the performance of LIBs. To meet the requirements of higher capacity and longer cycle life for LIBs, there has been an increase in studies focusing on the development of novel cathodes with higher working potentials [7–9]. However, the overcharge of LiCoO2 can provoke the serious oxidation of the electrolyte at higher potentials and cause a high- resistance cathodic film to form, thus causing the capacity fade of the LIBs in the following Copyright: © 2021 by the authors. Licensee MDPI, Basel, Switzerland. cycles [10–12]. Some side effects have been observed, including the dissolution of transition- This article is an open access article metal ions in the electrolyte and the reduced cycling stability of the cells [13]. It is well distributed under the terms and known that the conventional organic carbonate solvents have oxidization potentials of 5 V. conditions of the Creative Commons In addition, the oxidation reaction is catalyzed in the presence of transition-metal ions, and Attribution (CC BY) license (https:// the decomposition of electrolytes is accelerated at lower potentials, leading to unexpected creativecommons.org/licenses/by/ rapid capacity fading [14]. Ethers such as 1,3-dioxolane (DOL) and 1,2-dimethoxyethane 4.0/). (DME) also have high ionic conductivities and coulombic efficiencies [15,16]. However, Nanomaterials 2021, 11, 609. https://doi.org/10.3390/nano11030609 https://www.mdpi.com/journal/nanomaterials Nanomaterials 2021, 11, 609 2 of 16 ethers at typical salt concentrations of 0.1 or 1 M cannot be practically utilized because of their low oxidative stability (less than 4 V vs. Li/Li+)[16–18]. Sulfone-based high-voltage electrolytes with good oxidation resistance have low lattice energy, but their relatively greater wettability and higher viscosity can greatly affect the performances of LIBs [19]. For cell systems containing graphite negative electrodes, sulfone-based electrolytes are also restricted since the stable solid–electrolyte interface (SEI) at the graphite surface cannot be formed [20]. Compared with organic carbonate solvents, room-temperature ionic liquids (RTILs) have exhibited higher thermal stability, lower flammability and volatility, and wider electrochemical windows [21]. However, the compatibilities of these electrolytes are also unsatisfactory due to the low wettability. In addition, the relatively higher melting points have also been found to degrade their low-temperature performances [22]. Electrolytes with excellent electrochemical properties always play an important role in improving the stability of LIBs. However, researchers have had great difficulty in devel- oping novel electrolytes for high-performance LIBs. It is worth noting that the addition of a small amount of additive into the electrolyte is beneficial in forming a protective layer and preventing the solvent penetration; in this way, the possible damages to the electrode structure could be efficiently avoided. In addition, a film-forming additive for high-voltage cathode material in LIBs undergoes oxidation and decomposition reactions on the surface of the positive electrode, and a stable interface film is formed favoring the solvent system, thus reducing or preventing the further oxidation of the solvent sys- tem. Lithium bis(oxalato)borate (LiBOB) is a typical inorganic additive for high-voltage LIBs. Phosphides (such as tris(pentafluorophenyl)phosphine (TPFPP), tris(hexafluoro-iso- propyl)phosphate (HFiP) and N-(triphenylphosphoranylidene)aniline (TPPA)), sulfonate esters (such as methylene methanedisulfonate (MMDS)), carboxyl anhydrides (such as glu- taric anhydride and succinic anhydride), and fluorides (such as 1,1-difluoro-4-phenylbut-1- ene (DF)) exhibited similar surface-film-forming characters and could enhance the perfor- mance of high-voltage cathodes [23–29]. The oxidation of LiBOB on the cathode surface was found to generate a cathode passivation layer that inhibited the further oxidation of the electrolyte [30,31]. The complexing additives can form complexes with free transition-metal elements, purify the electrolyte system, suppress the electrolyte decomposition, and improve the high- voltage performance. For example, adiponitrile can inhibit the side reaction between the electrolyte and the surface of the high-nickel positive electrode, and the strong coordination between the nitrile group and Ni4+ can effectively reduce the formation of electrochemically inert NiO-type rock-salt structure [32]. Recently, dihydro-1,3,2-dioxathiolo[1,3,2]dioxathiole 2,2,5,5-tetraoxide (DDDT) has emerged as an efficient electrolyte additive for LIBs. DDDT has been utilized as an overall- functional electrolyte additive for high-voltage NCM523/graphite batteries, and enhanced electrochemical performance could be obtained [33]. In this study, to improve the interface stability of high-voltage lithium cobalt oxide (LiCoO2), DDDT-containing electrolyte was used as a multifunctional electrolyte additive. The physicochemical properties of the cells were analyzed, and the underlying mechanisms were investigated. In particular, the protective layers formed electrodes by the electrochemical redox reactions on the surfaces of both the cathode and the anode could greatly decrease the side reactions and improve the voltage stability of the electrodes. The research indicated that the DDDT-containing electrolyte was beneficial for the high-voltage LiCoO2 batteries, besides the previous breakthroughs towards LiNi0.5Co0.2Mn0.3O2/graphite batteries, which may provide a useful reference for the preparation of more stable LIBs by the formation of high-quality interfacial films in the cells. 2. Experimental Details 2.1. Materials, Electrolyte Configuration, and Cell Production Battery-level component LiPF6, ethylene carbonate (EC), propylene carbonate (PC), diethyl carbonate (DEC), ethyl methyl carbonate (EMC), n-propyl propionate (PP), and Nanomaterials 2021, 11, x FOR PEER REVIEW 3 of 18 2. Experimental Details 2.1. Materials, Electrolyte configuration, and Cell production Nanomaterials 2021, 11, 609 3 of 16 Battery-level component LiPF6, ethylene carbonate (EC), propylene carbonate (PC), diethyl carbonate (DEC), ethyl methyl carbonate (EMC), n-propyl propionate (PP), and dihydro-1,3,2-dioxathiolo[1,3,2]dioxathiole 2,2,5,5-tetraoxide (DDDT, Figure 1) were dihydro-1,3,2-dioxathiolo[1,3,2]dioxathioleprovided
Details
-
File Typepdf
-
Upload Time-
-
Content LanguagesEnglish
-
Upload UserAnonymous/Not logged-in
-
File Pages16 Page
-
File Size-