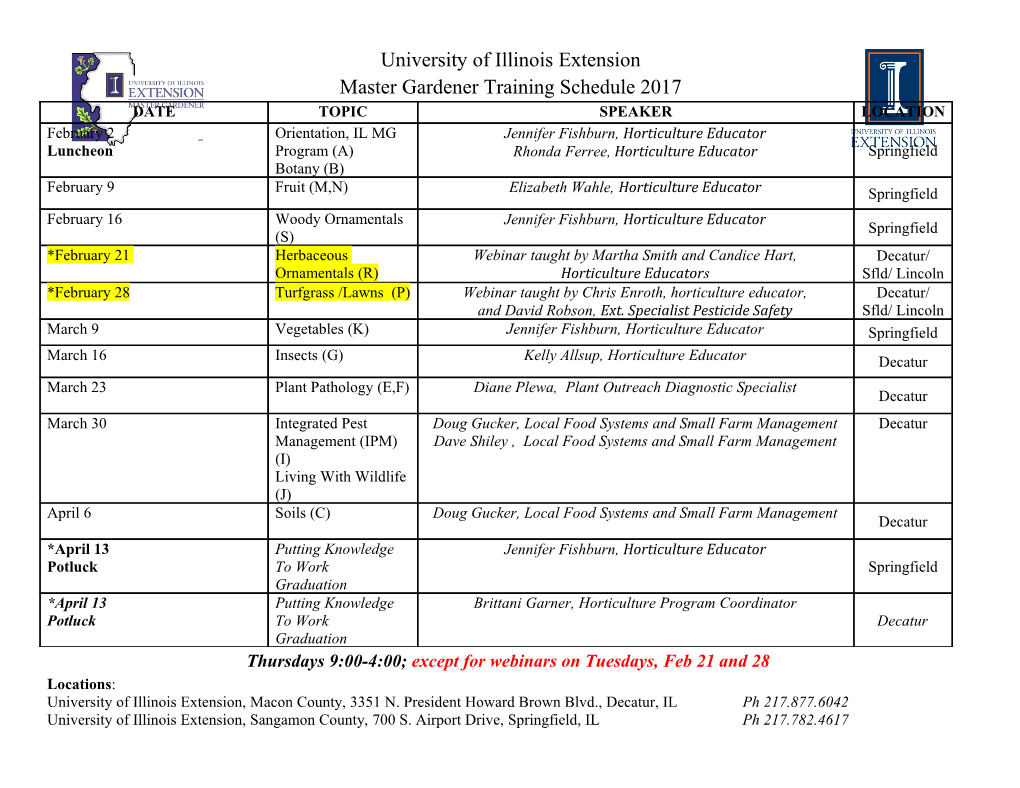
www.nature.com/npjcompumats REVIEW ARTICLE OPEN Plasmon-enhanced light–matter interactions and applications Huakang Yu1, Yusi Peng2,3, Yong Yang2,3 and Zhi-Yuan Li1 Surface plasmons are coherent and collective electron oscillations confined at the dielectric–metal interface. Benefitting from the inherent subwavelength nature of spatial profile, surface plasmons can greatly accumulate the optical field and energy on the nanoscale and dramatically enhance various light–matter interactions. The properties of surface plasmons are strongly related to materials and structures, so that metals, semiconductors and two-dimensional materials with various morphologies and structures can have alternating plasmonic wavelengths ranging from ultraviolet, visible, near infrared to far infrared. Because the electric field can be enhanced by orders of magnitude within plasmonic structures, various light–matter interaction processes including fluorescence, Raman scattering, heat generation, photoacoustic effects, photocatalysis, nonlinear optical conversion, and solar energy conversion, can be significantly enhanced and these have been confirmed by both theoretical, computational and experimental studies. In this review, we present a concise introduction and discussion of various plasmon-enhanced light–matter interaction processes. We discuss the physical and chemical principles, influencing factors, computational and theoretical methods, and practical applications of these plasmon-enhanced processes and phenomena, with a hope to deliver guidelines for constructing future high-performance plasmonic devices and technologies. npj Computational Materials (2019) 5:45 ; https://doi.org/10.1038/s41524-019-0184-1 INTRODUCTION SP IN DIFFERENT MATERIALS Over the past decades, surface plasmons (SPs) have attracted SPs originate from coupling between photons and free electrons. much attention due to its subwavelength spatial profile of modal Conventionally, SPs are investigated inside metals, such as noble field that can be harnessed to dramatically enhance light–matter metal gold and silver, with an abundant amount of free electrons 1–9 interactions. SPs are coherent and collective electron oscilla- and resonance wavelength located in the visible and near-infrared tions at the interface between two materials possessing positive regions. Later on, heavily doped semiconductors and 2D materials and negative real part of dielectric functions respectively (e.g., are also demonstrated to exhibit abundant plasmonic responses.10 metal–dielectric interface). SPs can be generally divided into two Usually the plasmon frequency ωp is given by categories, i.e., localized and propagating SP polaritons (SPPs). Benefited from the greatly enhanced local electric field, SP can π 2 1=2 remarkably enhance the interaction strength between photons ω ¼ 4 Ne ; p à (1) and materials, spurring the fast-growing developments of ε/m plasmon-enhanced fluorescence, Raman spectroscopy, heat gen- eration, photoacoustics, photocatalysis, nonlinear optics, solar energy conversion, and so on. where N is the density of carriers (electrons or holes), m* is the fi ω In this paper, we briefly review recent progress on plasmon- effective mass of carriers. One can easily nd that p is enhanced light–matter interactions by design of plasmonic proportional to square root of N. Figure 1 shows the value of ωp materials and structures. Firstly, we discuss SPs in metal, for various materials and nanoparticle sizes. For noble metal − semiconductor, and 2D materials with various morphologies, nanoparticles, typical value of N is 1022–1023 cm 3 and the structure regulations, and resonance wavelengths. Then we plasmon resonance wavelength is in the NIR and visible region. examine the principle of plasmon-enhanced light–matter interac- For doped semiconductors, typical value of N is 1016–1021 cm−3 tions in the scope of hot spots and discuss in more details several and the plasmon wavelength is in the THz and NIR region.11 As relevant theoretical and computational methods that enable one shown in Fig. 1, plasmon frequency can be tuned in a wide range to deeply understand these plasmon-enhanced phenomena and by simply changing free carrier or doping density. Aside from the processes. Next, we introduce several representative applications material properties, the plasmon resonance frequency and of plasmonic materials, structures, and devices such as plasmon- strength strongly depend on physical factors such as physical fl enhanced uorescence, Raman spectroscopy, heat generation, size, morphology, and geometrical arrangement. In the following photo-acoustics, photocatalysis, nonlinear optics, and solar cells. text we will discuss SPs in different materials with various Finally, we present summary and perspectives of plasmon- morphologies and structures. enhanced light–matter interaction. 1School of Physics and Optoelectronics, South China University of Technology, 510641 Guangzhou, China; 2State Key Laboratory of High Performance Ceramics and Superfine Microstructures, Shanghai Institute of Ceramics, Chinese Academy of Sciences, 1295 Dingxi Road, 200050 Shanghai, China and 3Graduate School of the Chinese Academy of Sciences, 100190 Beijing, China Correspondence: Yong Yang ([email protected]) or Zhi-Yuan Li ([email protected]) These authors contributed equally: Huakang Yu, Yusi Peng Received: 27 December 2018 Accepted: 8 March 2019 Published in partnership with the Shanghai Institute of Ceramics of the Chinese Academy of Sciences H. Yu et al. 2 Fig. 1 Schematic diagram of plasmon frequency dependence on free or doped carrier density for metals or semiconductors. (Reproduced with permission from ref. 11. Copyright Springer Nature 2011) SP in metal composed of multiple metallic structures, such as dimers and Metals are popularly assumed to be excellent candidates for aggregates, have been designed and fabricated to promote 9 plasmonic applications. Among them Ag is the best material for plasmonic enhancements to a very high level. Benefited from the plasmonics due to its lowest optical loss in the visible and NIR huge enhanced field inside the nanometer gap, two groups reported single-molecule Raman signal detection in Ag nanopar- 1234567890():,; spectral ranges. However, Ag would oxidize quickly and suffers severe losses due to surface roughness. Au is another popular ticle aggregates in 1997, with an estimated Raman enhancement 14 15 16,17 plasmonic material with excellent performance in the visible and factor up to 10 –10 . To relieve complex fabrication NIR spectral ranges, and superior chemical stability under ambient procedures, hybrid structures made from metallic nanoparticle conditions. As Ag and Au are very expensive, Cu and Al become placed on a metal substrate separated by a nanometer-thick alternative choices. However, Cu and Al both suffer from chemical dielectric thin film have been demonstrated to be efficient for instabilities under atmospheric conditions, restricting their further plasmonic enhancements. An alternative structure for efficient applications. Alkali metals are also ideal for plasmonic application, Raman enhancement is shown in Fig. 4, which are Ag sharp but they are so reactive to air and water that they must be stored nanoneedle arrays as fabricated by a simple Ar+-ion irradiation in vacuum or inert gas. Therefore, Alkali metals are rarely used for method and the Raman signal enhancement factor can reach plasmonics. Pd and Pt have attracted much attention owning to ~1010.18 Most recently, metallic nanoparticles hybridized with their applications in catalytic activities, although they are strongly magnetic materials have been successfully developed, where the absorptive in the visible wavelength and thus not good plasmonic plasmonic response of metallic nanorod can be adjusted by materials. Besides pure metals, alloyed metals are broadly simply changing the external magnetic field directions.19 considered for plasmonic applications. The alloying of different noble metals can be used to tune the SP resonance (SPR) SP in semiconductor wavelength accordingly. For example, AuxAg(1−x) alloy nanopar- – Heavily doped semiconductor materials exhibit metallic features ticles display SPR over a broad range of the UV vis spectra and and thus can be utilized for plasmonic applications. For heavily SPR wavelength exhibits red shift while increasing particle size. doped semiconductors (p- or n-type), the carrier density can be so The AuxAg(1−x) alloy nanoparticles with varying mole fractions high to exhibit metallic properties. For example, ReO3 type oxides, show only one plasmon absorption and similar plasmonic telluride or nitride materials have displayed extinct plasmonic strength as composite Gold and Silver. The plasmon band properties in the visible and NIR region. Similarly, plasmonic presents blue shift linearly with increasing mole fractions of 12–14 features in semiconductor could be easily modulated by changing silver. the shape, size, type, distribution of doped elements, and doping Another crucial aspect is to harness the geometric morpholo- concentration of materials.20 Considering distinct material proper- gies of metallic nanoparticles and nanostructures, which is ties of semiconductors from metals, SPs in semiconductors behave popularly explored so as to shape and manipulate plasmonic quite differently from SPs in metals. One promising feature of SPs properties. Figure 2 illustrates the calculation results of extinct, in heavily doped semiconductors is relatively low loss compared absorption,
Details
-
File Typepdf
-
Upload Time-
-
Content LanguagesEnglish
-
Upload UserAnonymous/Not logged-in
-
File Pages14 Page
-
File Size-