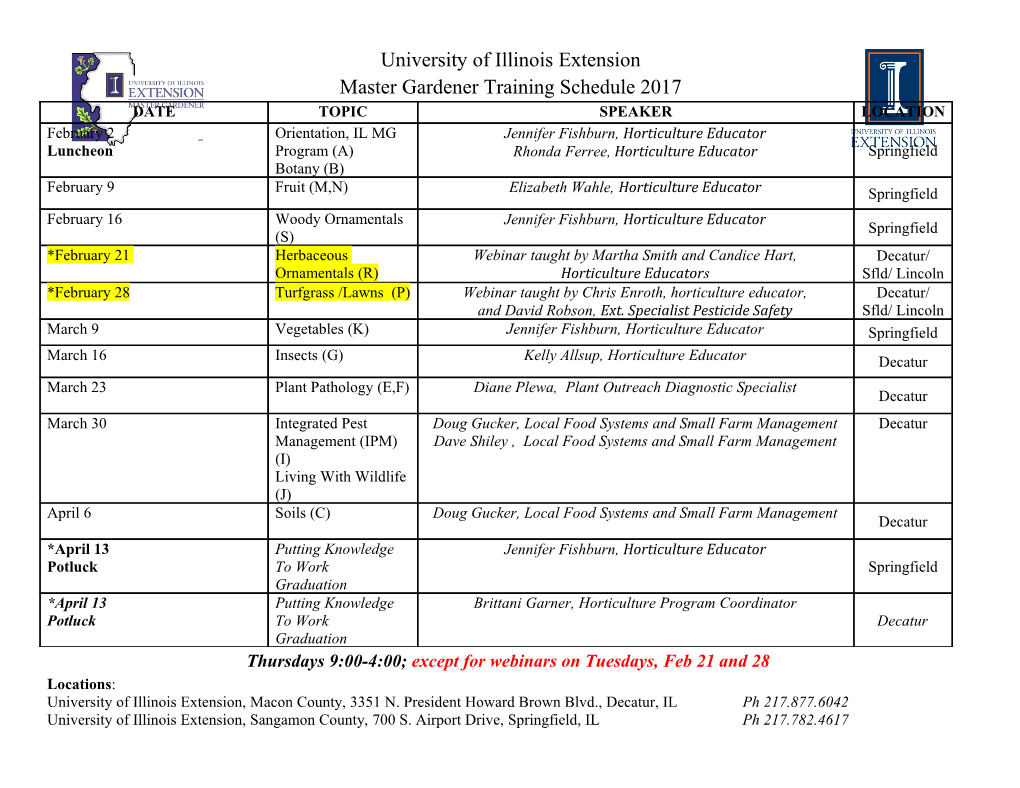
MNRAS 000,1{5 (0000) Preprint 9 January 2019 Compiled using MNRAS LATEX style file v3.0 Time-resolved image polarimetry of Trappist-1 during planetary transits P. A. Miles-P´aez1;2?, M. R. Zapatero Osorio3, E. Pall´e4;5, and S. A. Metchev1;6 1Department of Physics & Astronomy and Centre for Planetary Science and Exploration, The University of Western Ontario, London, ON N6A 3K7, Canada 2Steward Observatory and Department of Astronomy, University of Arizona, 933 N. Cherry Avenue, Tucson, AZ 85721, USA 3Centro de Astrobiolog´ıa(CSIC-INTA), Carretera de Ajalvir km 4, 28850 Torrej´onde Ardoz, Madrid, Spain 4Instituto de Astrof´ısica de Canarias, Calle V´ıaL´actea s/n, 38205 La Laguna, Tenerife, Spain 5Dpt. de Astrof´ısica, Univ. de La Laguna, Avda. Astrof´ısico Francisco S´anchez s=n, 38206 La Laguna, Tenerife, Spain 6Department of Physics & Astronomy, Stony Brook University, Stony Brook, NY 11794{3800, USA 9 January 2019 ABSTRACT We obtained linear polarization photometry (J-band) and low-resolution spectroscopy (ZJ-bands) of Trappist-1, which is a planetary system formed by an M8-type low-mass star and seven temperate, Earth-sized planets. The photopolarimetric monitoring cam- paign covered 6.5 h of continuous observations including one full transit of planet Trappist-1d and partial transits of Trappist-1b and e. The spectrophotometric data and the photometric light curve obtained over epochs with no planetary transits indi- cate that the low-mass star has very low level of linear polarization compatible with a null value. However, the \in transit" observations reveal an enhanced linear polariza- tion signal with peak values of p∗ = 0:1 % with a confidence level of 3 σ, particularly for the full transit of Trappist-1d, thus confirming that the atmosphere of the M8-type star is very likely dusty. Additional observations probing different atmospheric states of Trappist-1 are needed to confirm our findings, as the polarimetric signals involved are low. If confirmed, polarization observations of transiting planetary systems with central ultra-cool dwarfs can become a powerful tool for the characterization of the atmospheres of the host dwarfs and the validation of transiting planet candidates that cannot be corroborated by any other method. Key words: polarization { stars: atmospheres { stars: late-type { stars: low-mass { stars: individual: Trappist-1 1 INTRODUCTION ganized into \clouds", are expected to produce linear polar- ization at optical and near-infrared wavelengths via scat- The M8 star Trappist-1 is so far the only ultra-cool dwarf tering processes (Sengupta & Krishan 2001; de Kok et al. with transiting planets known (Gillon et al. 2016, 2017). It 2011). Observations reveal that linear polarization is mea- is thus a unique benchmark that may be used to explore the arXiv:1901.02041v1 [astro-ph.SR] 7 Jan 2019 surable in some late-M and L dwarfs, which show typical feasibility of new techniques for seeking and characterizing polarimetric degrees smaller than 1% in the I- and J-bands close-in exoplanets around very low-mass stars and brown (M´enard et al. 2002; Zapatero Osorio et al. 2005; Goldman dwarfs, which are typically too faint for precise radial veloc- et al. 2009; Zapatero Osorio et al. 2011; Miles-P´aez et al. ity measurements with current instrumentation. 2013, 2015, 2017). Because the net polarization of a purely Trappist-1 has an effective temperature T = 2516±41 eff spherical atmosphere is zero, the linear polarimetry detec- K(Van Grootel et al. 2018); this is low enough for naturally tions are usually explained by the asymmetries introduced forming liquid and solid condensates in the upper photo- by rotation-induced oblateness and/or patchy, non-uniform sphere (Fegley & Lodders 1996; Tsuji et al. 1996a,b; Jones distribution of atmospheric condensates. & Tsuji 1997; Allard et al. 1997, 2001). These condensates, sometimes referred to as \dusty" particles that can be or- Another likely asymmetry is the presence of a planet transiting the dwarf's dusty disk (Sengupta 2016, 2018). Ac- cording to the theory, the net non-zero, time-dependent po- ? E-mail: [email protected] larization is maximum at the inner contacts of the planetary c 0000 The Authors 2 P. A. Miles-P´aezet al. ingress and egress phases. The polarimetric light curve dur- tures using a ring with a inner radius of 6×FWHM and a ing the transit shows characteristic profiles depending on the width of 1×FWHM. We determined that apertures of 2{ planet-to-parent object size ratio and the orbital distance. 4×FWHM in size were adequate for measuring q and u. To Models also predict that the linear polarization caused by increase the signal-to-noise ratio (S/N) of the polarimetric planetary transits is higher in the J-band, and can be even data, we obtained the averaged q and u values for every 7 higher than what is produced by rapid rotation-induced independent, consecutive polarimetric measurements. Error oblateness. Here, we report on our J-band polarimetric mon- bars of q and u were then computedp as the standard devia- itoring of Trappist-1 during the full transit of planet d and tion of 7 measures divided by 7. We investigated the likely partial transits of planets b and e. relationship of the observed Stokes parameters with the air mass, FWHM, the X and Y position of the target centroid in each image, and the telescope rotator angle, but we did not find any obvious correlation (see AppendixB). The degree 2 OBSERVATIONS AND DATA ANALYSIS of linear polarization, P , and its associated uncertainty, σP , 2.1 Linear polarimetry imaging were derived from the quadratic sum of the Stokes parame- ters and their errors, respectively. For low values of P , and We collected polarimetry imaging data of Trappist-1 on the since P is always a positive quantity, the polarimetric de- night of 2017 September 29, when three transits (planets gree tends to be overestimated. We corrected for this effect b, d, and e) occurred. We employed the J-band filter cen- ∗ p 2 2 using p = P − σp (Wardle & Kronberg 1974; Simmons tered at 1.2508 µm (bandpass of 0.1544 µm) and the Long- & Stewart 1985), thus obtaining the debiased linear polar- slit Intermediate Resolution Infrared Spectrograph (LIRIS; +σ ization degree p∗ (p∗ = 0:0 p when σ > P ). Table A1 Manchado et al. 2004) attached to the Cassegrain focus of −0 p provides q, u, and p∗ along with their corresponding central the 4.2-m William Herschel telescope (WHT) on the Roque time and observing time interval. We did not compute the de los Muchachos Observatory. LIRIS is equipped with a vibration angle of polarization because the observed Stokes 1024×1024 HAWAII detector and has a plate scale of 000: 25 parameters are small and will lead to large angular uncer- pixel−1 projected onto the sky. This yields a field of view tainties. Instead, we shall describe the results of the polari- of 4:027 × 4:027. In polarimetric mode, two Wollaston prisms metric monitoring of Trappist-1 in terms of q, u, and p∗. split the light of the targets into four simultaneous images On the same observing night as Trappist-1, we also ac- corresponding to vectors 0, 90, 135, and 45 deg, each of them quired J-band linear polarimetry images of the unpolarized with a reduced field of view of 40 × 10 as shown in Figure A1 standard star HD 14069 (Turnshek et al. 1990) for control- in the AppendixA. We also observed our target using two ling the LIRIS instrumental polarization. Integrations of 4 different retarder plates (see Miles-P´aez et al. 2017), which s were employed per retarder plate. We obtained the fol- leads to a more precise determination of the polarization lowing values: q = 0:02 ± 0:04%, u = −0:03 ± 0:04%, and degree. p∗ = 0:00+0:06%, thus confirming that LIRIS has a very low As a result of our monitoring campaign, we obtained −0:0 instrumental polarization at J-band wavelengths, with an a total of 420 consecutive LIRIS frames on Trappist-1 (210 upper limit of 0.18% (3 σ confidence level). linear polarimetry measurements) over a continuous time period of ≈6.5 h. Individual integrations ranging from 20 through 70 s were applied depending on the air mass and seeing conditions. Because Trappist-1 is a bright M8 dwarf in 2.2 Linear spectropolarimetry the J-band (J = 11:35±0:02 mag) and in order to maximize We carried out low-resolution spectropolarimetry observa- the time cadence of the data, we performed stare observa- tions of Trappist-1 using LIRIS at the WHT on 2017 August tions (no nodding cycles). This observing strategy allowed us 4 between ≈4 UT and ≈5 UT, in which no planetary transits to maintain one reference star (2MASS J23063600{0502216, were expected to take place. This is about 7 weeks prior to Figure A1) within the LIRIS polarimetric field of view, which the monitoring of the planetary transits reported in section was convenient to construct the differential (intensity) light 2.1. In its spectropolarimetric mode, LIRIS is equipped with curve of Trappist-1 (Section 2.3). Raw data were reduced a narrow long slit that has 000: 75 width and 10 length. We using the Image Reduction and Analysis Facility software employed the low resolution ZJ grism. This instrumental (iraf1). Each polarimetric vector was flat-fielded separately configuration yields a wavelength coverage of 0.9{1.5 µm, using appropriate data acquired through the polarimetric a spectral resolution of 18.1 A(˚ R ≈ 600 at 1.1 µm), and optics and at very high air masses during dusk to avoid the a nominal dispersion of 6.06 A˚ pixel−1.
Details
-
File Typepdf
-
Upload Time-
-
Content LanguagesEnglish
-
Upload UserAnonymous/Not logged-in
-
File Pages8 Page
-
File Size-