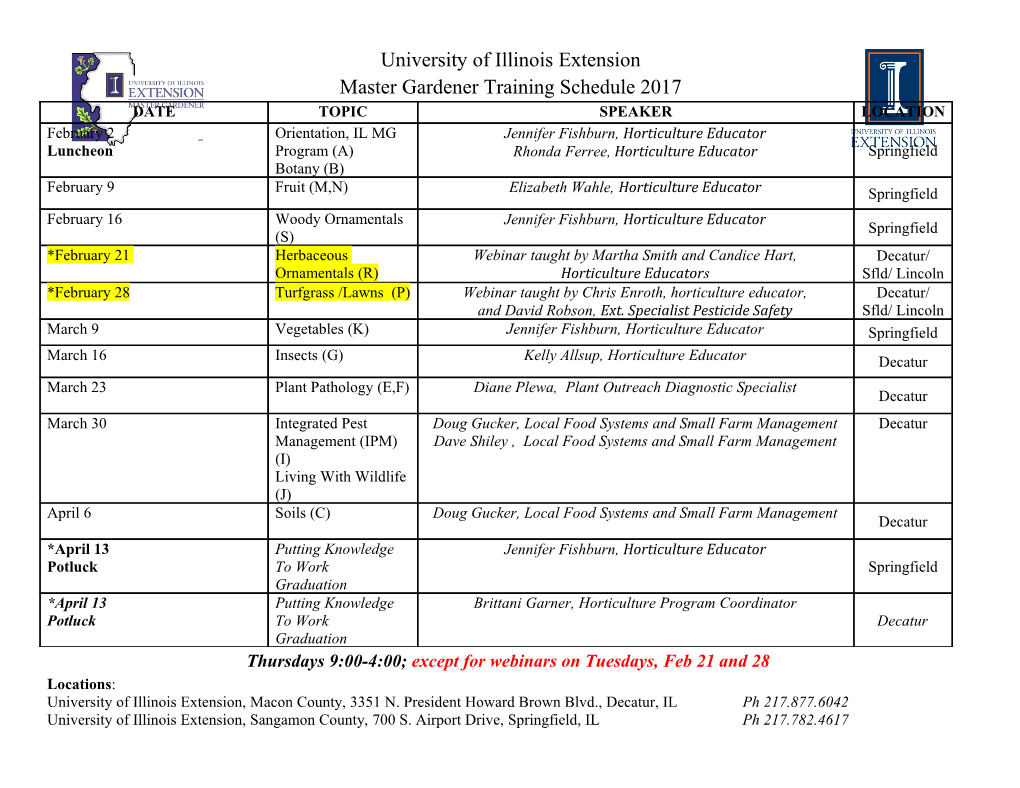
Architecture of the yeast elongator complex M Dauden, J Kosinski, Olga Kolaj-Robin, A Desfosses, A Ori, C Faux, N Hoffmann, O Onuma, K Breunig, M Beck, etal. To cite this version: M Dauden, J Kosinski, Olga Kolaj-Robin, A Desfosses, A Ori, et al.. Architecture of the yeast elon- gator complex. EMBO Reports, EMBO Press, 2017, 18 (2), pp.264-279. 10.15252/embr.201643353. hal-02289580 HAL Id: hal-02289580 https://hal.archives-ouvertes.fr/hal-02289580 Submitted on 16 Sep 2019 HAL is a multi-disciplinary open access L’archive ouverte pluridisciplinaire HAL, est archive for the deposit and dissemination of sci- destinée au dépôt et à la diffusion de documents entific research documents, whether they are pub- scientifiques de niveau recherche, publiés ou non, lished or not. The documents may come from émanant des établissements d’enseignement et de teaching and research institutions in France or recherche français ou étrangers, des laboratoires abroad, or from public or private research centers. publics ou privés. Article Architecture of the yeast Elongator complex Maria I Dauden1, Jan Kosinski1, Olga Kolaj-Robin2,3,4, Ambroise Desfosses1,†, Alessandro Ori1,‡, Celine Faux2,3,4,§, Niklas A Hoffmann1, Osita F Onuma5, Karin D Breunig5 , Martin Beck1 , Carsten Sachse1 , Bertrand Séraphin2,3,4, Sebastian Glatt6,* & Christoph W Müller1,** Abstract chaperones [1–3]. Previous studies indicated that specific base modifications in the wobble base position of tRNAs are crucial to The highly conserved eukaryotic Elongator complex performs maintain these highly dynamic and complex mechanisms. Mainly specific chemical modifications on wobble base uridines of tRNAs, because they influence the recognition rate and affinity between which are essential for proteome stability and homeostasis. The incoming tRNAs and codons in the A site of the translating ribo- complex is formed by six individual subunits (Elp1-6) that are all some [4–6]. Interestingly, expansion of tRNA gene copy numbers equally important for its tRNA modification activity. However, its and isoacceptors correlate well with an increase in different tRNA overall architecture and the detailed reaction mechanism remain modification enzymes, suggesting an evolutionary selection for elusive. Here, we report the structures of the fully assembled yeast optimizing translational efficiency and accuracy via different modi- Elongator and the Elp123 sub-complex solved by an integrative fication mechanisms [7]. structure determination approach showing that two copies of the The eukaryotic Elongator complex has been associated with a Elp1, Elp2, and Elp3 subunits form a two-lobed scaffold, which plethora of cellular activities [8–10], but nowadays, it is widely binds Elp456 asymmetrically. Our topological models are consis- accepted that the main cellular function of the Elongator complex is tent with previous studies on individual subunits and further vali- the formation of 5-methoxycarbonylmethyl-uridine (mcm5U), 5- dated by complementary biochemical analyses. Our study provides methoxycarbonylmethyl-2-thio-uridine (mcm5s2U), and 5-carba- a structural framework on how the tRNA modification activity is moylmethyl-uridine (ncm5U) in the wobble base position of 11 carried out by Elongator. eukaryotic tRNAs [10]. Nevertheless, the detailed chemistry of the Elongator modification reaction is insufficiently described, and the Keywords electron microscopy; Elongator; Saccharomyces cerevisiae; tRNA role of the resulting modifications is not fully understood [11–13]. modification; yeast In particular, it is currently unclear how tRNA is delivered to the Subject Categories RNA Biology; Structural Biology active center and how the high modification turnover can be DOI 10.15252/embr.201643353 | Received 15 September 2016 |Revised20 achieved in the context of the full complex. The cellular role of Elon- October 2016 | Accepted 8 November 2016 | Published online 14 December 2016 gator is of fundamental clinical relevance, as mutations affecting the EMBO Reports (2017) 18: 264–279 integrity and activity of this macromolecular complex are related to the onset of neurodegenerative diseases [14–16], cancer [17,18], See also: DT Setiaputra et al and intellectual disabilities [19]. The fully assembled Elongator complex contains two copies of each of its six subunits in vivo [20] and has an estimated molecular Introduction weight of ~850 kDa. All subunits are highly conserved among eukaryotes [21], which has also been experimentally proven by During the elongation phase of the ribosome-mediated translation cross species complementation analyses of genes encoding individ- process, transient pausing events support proper domain folding ual subunits and subdomains for yeast, insects, worms, plants, and of the nascent polypeptide chains, which gain their three- humans [15,22–26]. Shortly after the initial description of the three- dimensional conformations immediately after they have left the component Elongator sub-complex (Elp123) [27], an additionally exit tunnel of the ribosomes, a process sometimes facilitated by associated sub-complex containing subunits Elp4, Elp5, and Elp6 1 European Molecular Biology Laboratory, Structural and Computational Biology Unit, Heidelberg, Germany 2 Université de Strasbourg, IGBMC, Illkirch, France 3 CNRS, IGBMC UMR 7104, Illkirch, France 4 Inserm, IGBMC U964, Illkirch, France 5 Institute of Biology, Martin Luther University Halle-Wittenberg, Halle (Saale), Germany 6 Max Planck Research Group at the Malopolska Centre of Biotechnology, Jagiellonian University, Krakow, Poland *Corresponding author. Tel: +48 12 664 6321; E-mail: [email protected] **Corresponding author. Tel: +49 6221 387 8320; E-mail: [email protected] †Present address: School of Biological Sciences, University of Auckland, Auckland, New Zealand ‡Present address: Leibniz Institute on Aging-Fritz Lipmann Institute, Jena, Germany §Present address: CRBM – CNRS UMR5237, Montpellier, France 264 EMBO reports Vol 18 |No2 | 2017 ª 2016 The Authors. Published under the terms of the CC BY 4.0 license Maria I Dauden et al Structural characterization of yeast Elongator EMBO reports [28] was identified under milder purification conditions. Crystal The Elp456 ring is asymmetrically positioned in the structures are currently available for the homo-dimer of the Elp1 C- holoElongator complex terminus [29], Elp2 [30], and the RecA-like Elp456 hetero-hexamer [20,31]. We recently determined the crystal structure of full-length In order to obtain the structure of Elongator, we used negative-stain Elp3 from a bacterial homolog (DmcElp3), which shows high EM of the purified Elp6-TAP holoElongator complex. Highly pure sequence similarity to Elp3s from different organisms, including and stoichiometric holoElongator complex (Fig 1A) was stabilized yeast and humans [32]. The structure shows that the lysine acetyl using low amounts of glutaraldehyde and reapplied to gel filtration. transferase (KAT) domain and the S-adenosylmethionine binding A homogeneous population of two-lobed particles was observed domain (SAM) share a large and highly conserved interface that (Fig 1B) and 22,876 particles were selected and subjected to refer- creates a specific tRNA binding pocket and forms a composite active ence-free two-dimensional (2D) classification. The class averages site. However, no structural information is yet available for the show a complex of around 260 × 170 A˚ formed by two lobes with a Elp123 sub-complex or the holoElongator (Elp1-6), precluding the cleft in between (Fig 1C). Interestingly, a hexameric ring-shaped localization of the active center in relation to the other complex density protruding from one of the lobes is clearly visible (Fig 1C). components. In addition, some of the side view 2D averages are asymmetric, We set out to obtain structural information on the fully assem- while some top views do not show a ring. Together, these observa- bled Elongator complex to better understand how the different sub- tions suggest that only one copy of the hetero-hexameric Elp456 ring units interact and together deliver modifiable tRNAs to the asymmetrically interacts with the Elp123 sub-complex, as previ- enzymatically active Elp3 subunit. Here, we report the structures of ously described [20]. holoElongator and the Elp123 sub-complex at 31 A˚ and 27 A˚ resolu- The structure of holoElongator (Fig 1D) is ~270 A˚ in height, tion, respectively, by negative-stain electron microscopy (EM). In ~180 A˚ length, and ~160 A˚ width, and there is a clear correlation addition, we describe the global interaction network between all six between the class averages and the model back-projections (Fig 1C). individual subunits using crosslinking mass spectrometry (XL-MS) The Elongator model shows an estimated resolution of 31 A˚ based and combine these results by an integrative modeling approach, on the Fourier Shell Correlation (FSC; Fig EV2A), and although the which allows us to localize all subunits and provide a topological angular assignment of particle orientations is well distributed, we model of the full complex. The model enables us to anticipate how observed a preferential orientation, a commonly observed phenom- the tRNA modification activity is carried out by this large molecular enon in negative-stain EM (Fig EV2B). The structure consists of two machine and how the individual subunits contribute to its assembly lobes linked in the upper part by the “arch” and divided by a “cleft”, and activity. with two lateral bean-shaped densities, herein
Details
-
File Typepdf
-
Upload Time-
-
Content LanguagesEnglish
-
Upload UserAnonymous/Not logged-in
-
File Pages17 Page
-
File Size-