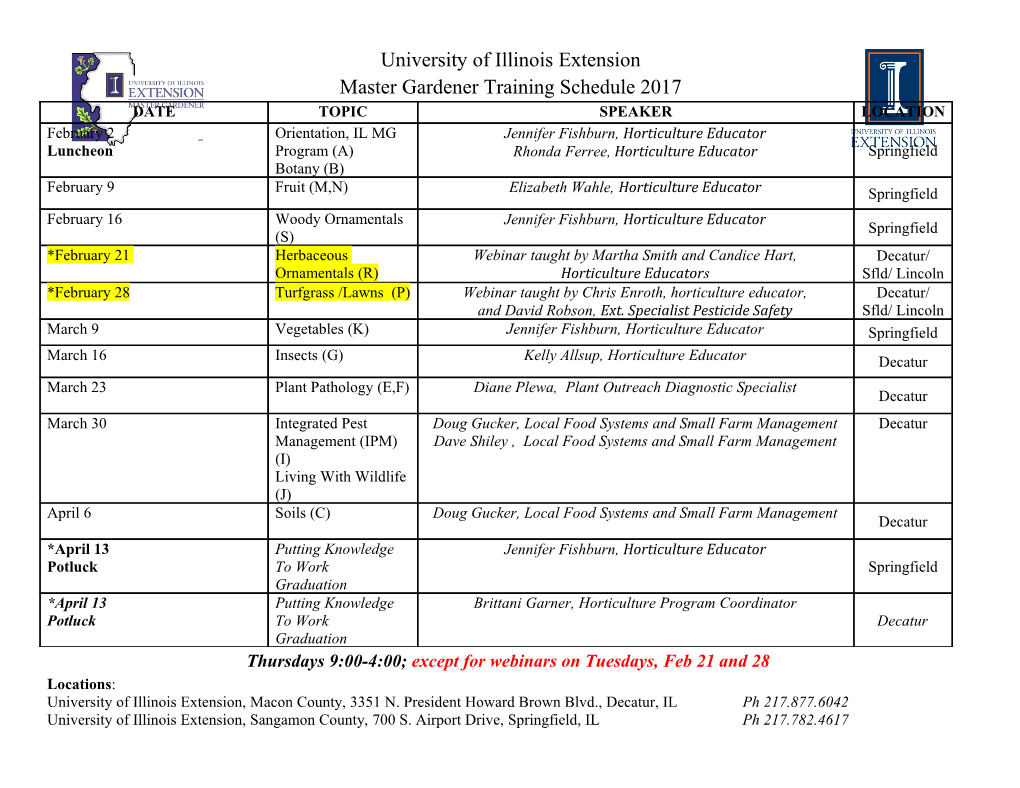
Atmosphere-surface vapor exchange and ices in the Martian polar regions Dissertation zur Erlangung des Doktorgrades der Mathematisch-Naturwissenschaftlichen Fakultaten¨ der Georg-August-Universitat¨ zu Gottingen¨ vorgelegt von Ganna Valeriyivna Portyankina aus Karachevka/Ukraine Gottingen¨ 2005 Bibliografische Information Der Deutschen Bibliothek Die Deutsche Bibliothek verzeichnet diese Publikation in der Deutschen Nationalbibliografie; detaillierte bibliografische Daten sind im Internet uber¨ http://dnb.ddb.de abrufbar. D7 Referent: Dr. U. Christensen Korreferent: Dr. H.U. Keller Tag der mundlichen¨ Prufung:¨ 12 September 2005 Copyright °c Copernicus GmbH 2006 ISBN 3-936586-47-0 Copernicus GmbH, Katlenburg-Lindau Druck: Schaltungsdienst Lange, Berlin Printed in Germany Contents Summary 5 1 General Introduction 7 1.1 History of Martian polar observations . 7 1.1.1 The first detection of polar caps and early observations . 7 1.1.2 More recent telescopic observations . 8 1.1.3 Spacecraft observations of polar regions and ices on Mars . 9 1.2 Martian polar caps and their role in the martian climate and evolution . 11 1.3 Differences between South and North polar caps . 12 1.4 Outline . 17 2 Spider patterns in the Martian cryptic region - observational data 19 2.1 Cryptic region and CO2 slab ice . 19 2.1.1 Cryptic region detection . 19 2.1.2 Optical properties of CO2 ice and solid-state green-house effect . 21 2.2 Spiders in MGS MOC images . 24 2.2.1 MOC polar observations . 24 2.2.2 Spider patterns in MOC narrow angle images . 26 2.2.3 Spatial distribution of spiders. Are all spiders inside the cryptic region? . 29 2.2.4 Fan structures, winds and seasonal distribution . 30 2.3 MOLA data of spider areas . 32 2.4 Conclusions . 36 3 Modeling of spiders 37 3.1 Formation of spiders . 37 3.2 Simulations of CO2 ice cleaning process . 39 3.2.1 Insolation of the South polar region . 39 3.2.2 Single particle modeling . 40 3.2.3 Dust inside an ice slab . 47 3.2.4 Net effect . 52 3.2.5 Energy flux . 53 3.3 Simulations of geyser-type eruptions and CO2 slab ice rigidity . 57 3.3.1 Calculations of stresses inside CO2 ice plate . 57 3.3.2 Pressure below ice plate . 60 3.3.3 Discussion of material strength . 61 3 Contents 3.4 Conclusions . 63 4 Seasonal evolution of the Martian cryptic region: influence of the atmospheric opacity 65 4.1 Introduction . 65 4.2 Observations . 67 4.3 Model description and results . 74 4.3.1 Model description . 74 4.3.2 Model results . 74 4.4 Conclusions . 79 5 Lee wave clouds in the Martian atmosphere: observations by HRSC 81 5.1 Observations of Martian lee-waves before HRSC . 81 5.2 Wind velocity measurement on Mars . 82 5.2.1 Direct measurements of wind velocities on Mars . 82 5.2.2 Winds derived from the cloud motion. 83 5.2.3 Winds derived from modeling or analysis of temperature fields. 83 5.3 Mars Express (MEX) and the High Resoluting Stereo Camera (HRSC), an overview . 84 5.4 The formation of lee wave clouds - mathematical formulation . 85 5.5 Measuring wind velocities from HRSC images . 91 5.6 Conclusions . 92 A Mars Global Surveyor MOC images which contain spiders patterns. 97 Bibliography 107 Acknowledgements 113 Lebenslauf 115 4 Summary This thesis is a report of my quest for understanding a few of the many processes that shape the Martian polar regions into the wide variety of landforms that have been observed with various spacecraft during recent years. The main part of this work is dedicated to the study of relatively small (10m-1km) features in the South polar regions called spider patterns. We collected observational data available from Mars Global Surveyor instruments (MOC, TES, and MOLA) of areas where spiders were found. We modeled spider formation on basis of the scenario proposed by Kieffer (2003). We checked two stages of Kieffer’s scenario: first, the cleaning of CO2 slab ice from dust, and then, the breaking of the slab ice plate under the pressure built below it by subliming ice. Our modelling indicates that spherical grains can reach the bottom of a 1 m thick layer of CO2 ice in a relatively short time of less then 20 days. During the beginning of spring, the dust sinking rate is higher than the ice sublimation rate at the top of the slab. Spherical dust grains can sink so fast that the CO2 ice becomes completely clean of dust. If the dust particles are randomly oriented thin disks, then only the upper part of ice will be cleaned. At a latitude of 75±S it will take between 1 and 20 days to build enough pressure below a slab to break it. The plate should break before ± Ls = 175 . Our results support scenario proposed by Kieffer. Spider patterns are found in the so called cryptic region. Part of the cryptic region significantly changed its appearance between 2001 and 2003. This change may be related to the global dust storm of 2001. We modeled the seasonal ice sublimation/condensation cycle to show that the evolution of this area of the cryptic region was affected by the dust storm during the year 2001. The model includes self-consistent treatment of sublimation and condensation of CO2 and H2O ices, and was used to calculate surface temperatures and the thicknesses of CO2 and H2O ice layers during these two years. Our modelling shows that the dust storm lowered surface temperatures in spring, and thus caused later than usual seasonal sublimation of both CO2 and water ices. The dust storm also consid- erably decreased the surface albedo. These two important effects almost cancel: the solar flux is reduced during the dust storm, but at the same time the dust that precipitates onto the surface reduces the albedo. Thus, the surface absorbed a bigger fraction of the solar radiation. The surface temperature stayed at about 146K for almost half of the Martian year, both during 2001 and 2003. We also studied the influence of surface roughness. We used images of lee wave clouds acquired by HRSC to infer the velocity of the driving wind. Wind speeds have only rarely been measured on Mars, although knowledge about them is essential for understanding the local climate and evolution. HRSC images taken during orbits 68, 719, 751, 1096 show lee wave clouds distinct enough to measure their wavelength. We infer wind speeds of 24.5 - 25.2 m s¡1 from these images. 5 1 General Introduction 1.1 History of Martian polar observations 1.1.1 The first detection of polar caps and early observations The polar ice caps are the most conspicuous albedo features on the surface of Mars, there- fore it is not surprising that they were among the first features to be identified on the Mar- tian surface. During 1659 Christiaan Huygens was the first to detect albedo features on the planet. Three martian oppositions later, during 1666, Giovanni Cassini made about twenty sketches of Mars at the observatory in Bologna. He was apparently the first to notice bright markings at Mars’ poles. Probably this was the discovery of the polar caps. Since then they have been observed often during more then two centuries by terrestrial observers and with instruments onboard many spacecrafts. One of the sketches by Huygens in 1672 shows the South cap. Maraldi made obser- vations during every opposition from 1672 to 1719, and he was lucky in that Mars came closer to the Earth in 1719 than it would for another 284 years. Maraldi described both polar caps but refrained from calling them ice-caps, and merely wrote about ”white spots”. He also discovered that the center of the South cap is not at the rotational pole, reported about temporal changes of the cap in connection with with equatorial dark areas, and described a dark band around the edge of one cap, which he interpreted as melted water. Frederick William Herschel, between 1777 and 1784, was the first to suggest the polar caps consisted of ice and snow that grow and shrink with the season. He observed Mars during the oppositions from 1777 to 1783 and reported on this in a paper called ”On the remarkable appearances at the polar regions of the planet Mars, the inclination of its axis, the position of its poles, and its spheroidal figure; with a few hints relating to its real diameter and atmosphere”. Hershel estimated the inclination of Mars’ equator to its orbit plane at around 30±, and thought the seasons on Mars to be similar to the terrestrial ones. He speculated that the polar caps are thin layers of ice and snow. Nicolas Camille Flammarion summarized the knowledge about Mars in a two-volume tome. The first volume was published in 1892 and had the following notes about obser- vations of the polar regions before 1830 (from Kieffer et al. (1992)): ”There are also on Mars white spots, marking its poles. These spots vary with season, increasing in winter, diminishing in summer. They submit to the influence of the Sun like our polar ice. We can consider them to be ice or snow.” ”These polar snows are not situated exactly at the extremities of the same diameter and do not mark absolutely the geographic poles. These poles are generally covered. But, 7 1 General Introduction at the epoch of minimum, they are reduced to a white point approximately circular that is removed a certain distance from the pole” 1.1.2 More recent telescopic observations During the first half of the 20th century, all possible technics of observational astron- omy (visual, photographic, spectrometry, radiometry and polarimetry) were used to study Mars.
Details
-
File Typepdf
-
Upload Time-
-
Content LanguagesEnglish
-
Upload UserAnonymous/Not logged-in
-
File Pages115 Page
-
File Size-