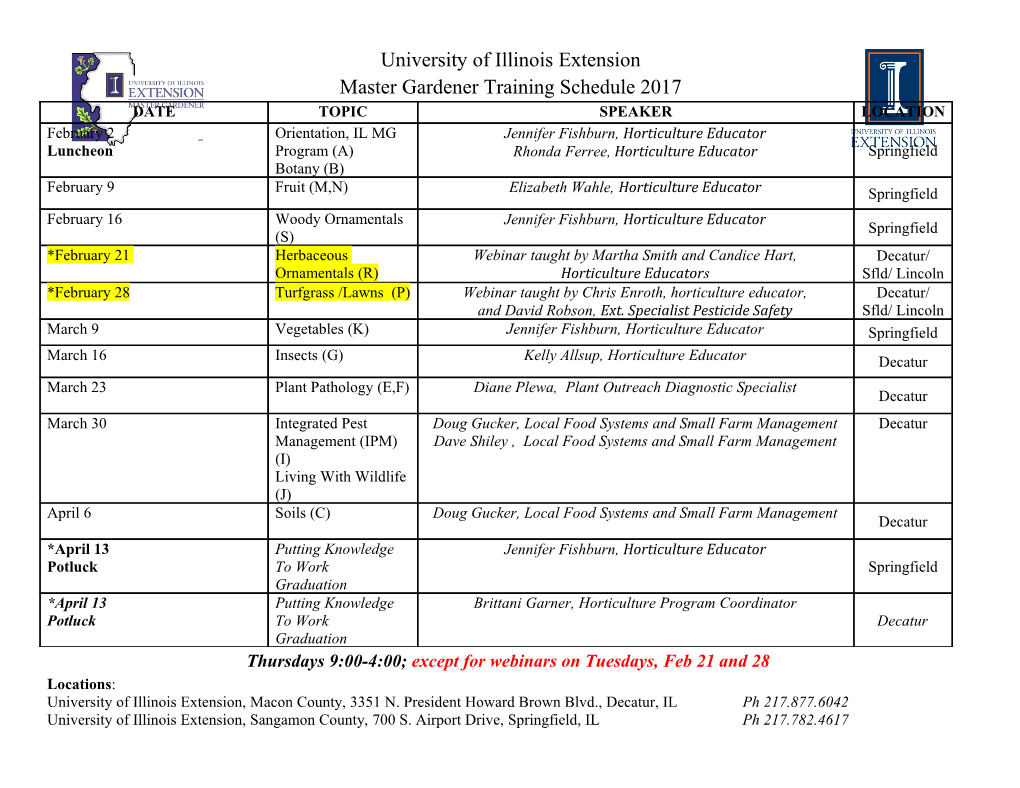
IAA COMMISSION III SG 2 – NUCLEAR SPACE POWER AND PROPULSION M. Auweter-Kurtz C. Bruno D. Fearn H. Kurtz T.J. Lawrence R.X. Lenard List of contents Introduction 8 1. Physics of Nuclear Propulsion – An Introduction 11 1.1. ABSTRACT 11 1.2. Introduction 11 1.3. Fundamental Physics 12 1.3.1. Forces 12 1.4. Propulsion 19 1.4.3. Power 23 1.4.4. Mass 25 1.5. Nuclear Propulsion Strategies 27 1.5.1. Nuclear Thermal Rockets (NTR) 27 1.5.2. Nuclear Electric Propulsion (NEP) 31 1.5.3. A Comparison between Chemical and NTR/NEP Isp 32 1.6. Massless (Photonic) Propulsion 33 1.7. Conclusions 34 1.8. References 35 2. Nulcear Thermal Rocket Propulsion Systems 38 2.1. ABSTRACT 38 2.2. Introduction 38 2.3. System Configuration and Operation 41 2.4. Particle-Bed Reactor 46 2.4.1. CERMET 47 2.5. Safety 49 2.6. MagOrion and Mini-MagOrion 51 2.7. Conclusions 53 2.8. References 54 3. The application of ion thrusters to high thrust, high specific impulse nuclear-electric missions 57 3.1. ABSTRACT 57 3.2. Introduction 60 3.3. Background 62 3.3.1. Space Nuclear Programmes 62 2 3.3.2. Advantages of Electric Propulsion 63 3.3.3. Propulsion System Parameters 64 3.3.4. Propulsion Technology Review 66 3.3.4.1. Gridded Ion Engines 66 3.3.4.2. The Hall-Effect Thruster 69 3.3.4.3. Magnetoplasmadynamic (MPD) Thrusters 71 3.3.4.4. Variable Specific Impulse Magnetoplasma Rocket (VASIMR) 72 3.4. Gridded Ion Engines; Current Devices 73 3.4.1. Ionization Mechanisms 73 3.4.1.1. Radiofrequency Discharge Thrusters 73 3.4.1.2. Radiofrequency Discharge Thrusters Operating at High Frequencies. 75 3.4.1.3. Kaufman-Type Direct Current Discharge Thrusters 76 3.4.1.4. Magneto-electrostatic Containment (MESC) DC Thrusters 77 3.4.2. Current Gridded Thrusters 79 3.4.3. Summary of Capabilities of Existing Gridded Thrusters 90 3.5. The Scaling Process 93 3.5.1. Grid Design Options 93 3.5.2. Exhaust Velocity and SI 96 3.5.3. Thrust and Perveance 97 3.5.4. Current, Power and Thrust Densities 98 3.5.5. The Discharge Chamber 99 3.6. High SI, High Power Operation 100 3.6.1. Parametric Variations 100 3.6.2. Operation at 100s of kW Level 101 3.6.2.1. Increase of Thruster Diameter 101 3.6.2.2. Increase of SI 102 3.6.2.3. Increase of Perveance 103 3.6.2.4. Technology Readiness 104 3.6.3. Operation at the MW Level 105 3.6.3.1. Specific Designs at the MW Level 107 3.6.3.2. Peak Thruster Performance 107 3.6.3.3. Propellant Selection 108 3.6.3.4. Parameter Ranges 109 3.6.4. Systems Considerations 110 3 3.7. Conclusions 111 3.8. References 114 3.9. List of Symbols and Acronyms 123 4. HIGH POWER AND HIGH THRUST DENSITY ELECTRIC PROPULSION FOR IN-SPACE TRANSPORTATION 125 4.1. Abstract 125 4.2. Introduction 125 4.3. Thermal Arcjets 126 4.3.1. Operational Principle 126 4.3.1.1. Efficiency. 127 4.3.1.2. Discharge Voltage. 129 4.3.2. Theory and Numerics 129 4.3.3. System Description 130 4.3.4. Influence of Propellants 131 4.3.5. Lifetime Limiting Factors 132 4.3.6. Qualification Advantages 133 4.3.7. Existing Arcjet Thruster Technologies 133 4.3.7.1. USA 133 4.3.7.2. Germany 133 4.4. Magnetoplasmadynamic Thruster 134 4.4.1. Self-Field (SF-MPD) Thrusters 135 4.4.1.1. Importance of Electrode Design. 141 4.4.1.2. Electrode Voltage Drops and Loss Distribution. 142 4.4.1.3. Electrode Erosion. 144 4.4.1.4. Electrode Losses and Cooling. 145 4.4.2. Magnetoplasmadynamic Thruster with Coaxial Applied Field 148 4.4.2.1. Acceleration Mechanism. 148 4.4.2.2. Hall Parameter. 149 4.4.2.3. Current Distribution 149 4.4.2.4. Movements of Charged Particles in E and B Fields. 150 4.4.2.5. Rotational Frequency of the Plasma. 151 4.4.2.6. Thrust. 152 4.4.2.7. Experimental Evidence. 153 4.4.2.8. Applicable Propellants. 155 4 4.4.2.9. Numerical Simulation. 155 4.4.2.10. Thruster Developments. 156 4.4.2.11. Necessary Test Facilities 158 4.5. High Power Hybrid Thruster Concept ATTILA 159 4.6. Summary 160 4.7. References 161 5. A review of reactor configurations for space nuclear electric propulsion and surface power considerations 167 5.1. ABSTRACT 167 5.2. Reactor introduction 167 5.3. Reactor REQUIREMENTS 168 5.4. Reactor And Power Conversion - Mass Comparison 169 5.4.1. Earlier Work and Reactor Descriptions 170 5.4.2. Static Model Development and Validation 172 5.4.2.1. Model Basis and Overview 172 5.4.2.2. Specific Design Anchor Points – Level Field. 173 5.4.2.3. Model Validation and Results. 181 5.4.3. A Simple Shield Model 183 5.4.4. Shield Parametrics 186 5.5. Reactor operation startup 189 5.6. Summary 190 5.7. References 191 6. Nuclear Safety, legal aspects and policy recommendations 192 6.1. ABSTRACT 192 6.2. Finding 1: Nuclear power and energy have significant roles in space exploration now, and the future for nuclear power has exceptional potential for future space exploration activities. 192 6.3. Finding 2: In order for the great potential advantages of nuclear propulsion to be realized, it must be perceived by a majority of the population to be safe. 193 5 6.4. Finding 3: Existing policies and procedures are generally adequate to account for requirements of public safety and environmental compliance. Some recommendations will assist in clarifying the meaning of some of these procedures, principles and policies to aid in the space systems engineering process. 194 6.4.1. History, Perspectives and Objectives 194 6.4.2. Safety Guidelines and Implementation. 195 6.5. Finding 4: The existing design, fabrication and test process, including safety analyses is adequate for addressing all non-launch related safety and environmental issues for a space nuclear reactor system; launch and space related protocols must be developed. 201 6.5.1. Recommendation 16: The Space Nuclear Reactor Program Should Concentrate on Major Post-Shipment Activity and Accident Categories 202 6.5.2. Arrival at Launch Site: Possible Scenarios 203 6.5.3. Spacecraft Final Assembly and Checkout: Possible Scenarios 203 6.5.4. Launch Preparation and Countdown: Possible Scenarios 204 6.5.5. Early Launch Phase: Possible Scenarios 205 6.5.6. Late Launch Phase: Possible Scenarios 205 6.5.7. Finding 5: The Safety and Operations Phase for NEP or NTP Systems Should be Developed so as to Maximize Possible Scenarios for Space Nuclear Reactor Employment 206 6.5.8. Recommendation 17: Hazards and Risks Definitions Should be Presented in a Consistent Fashion Throughout the Space Nuclear Reactor Program 207 6.5.9. Scenario Bins From Cassini FSAR Format 208 6.5.10. Conversion of Hazard Categories From Cassini FSAR Format To Hazards Table Format 209 6.5.11. Failure Events That Could Result In Release Of Radiation/Nuclear Material 210 6.6. Finding 7: Safety Assessment for Additional Risks Posed By Lunar and Mars Base Mission Scenarios Indicate that Space Reactor Systems can be used Safely and Effectively on the Surfaces of Other Celestial Bodies 211 6 6.6.1. Finding 8: A definable set of hazards for a surface nuclear reactor power system can be delineated and risks can be effectively mitigated 212 6.6.2. Primary Differences Between Cargo and Robotic Payload NEP system and a Moon or Mars Base Power System that can Impact Safety 213 6.6.2.1. Potential Safety Issues Associated with the Mars Base Power System 213 6.6.2.2. Initiating Events for Mars Base Power System 214 6.7. Finding 9: There appears to be no reason that a space nuclear reactor power system cannot be safely deployed and operated on the surface of another world while maintaining standards of planetary protection. 216 6.8. Finding 10: A Space Reactor System Enables Effective of Design Options in Mitigating Potential Radiation Releases 216 6.9. Finding 11: A Transparent and Systematically Traceable Space System Safety Test and Analysis Program Must be Conducted to Ensure Crew and Public Safety 216 6.9.1. Safety Testing 217 6.9.2. Propellant Explosion and Fire Tests 217 6.9.3. Impact Tests 218 6.9.4. Reentry Tests 219 6.9.5. Launch Environment Tests 219 6.9.6. Criticality Tests 220 6.10. Finding 11: Prior Space Reactor Programs Expended Resources on Destructive Disassembly Testing for Low-Probability Incidents – System Level Testing Should be Reserved for More Likely Scenraios 220 6.10.1. Safety Analysis 221 6.10.2. Neutronics 221 6.10.3. Shielding 221 6.10.4. Fires and Explosions 221 6.10.5. Intentional Disassembly 221 6.10.6. Reentry Analysis 222 6.10.7. Coupled Impact/Reactivity Analyses 222 6.10.8. Risk/Consequence Analysis 223 6.10.9. Other Analysis 224 7 6.11. Finding 19: An Integrated Approach for Performance and Safety Analysis and Testing is Critical to a Cost-Effective Development Program 225 6.11.1. Safety Program Organization 225 6.12. Finding 20: The Ultimate Objective of All Programmatic Activities is to Obtain Launch Approval for the Space Reactor System – the Program Should be Structured to Attain the Goal.
Details
-
File Typepdf
-
Upload Time-
-
Content LanguagesEnglish
-
Upload UserAnonymous/Not logged-in
-
File Pages257 Page
-
File Size-