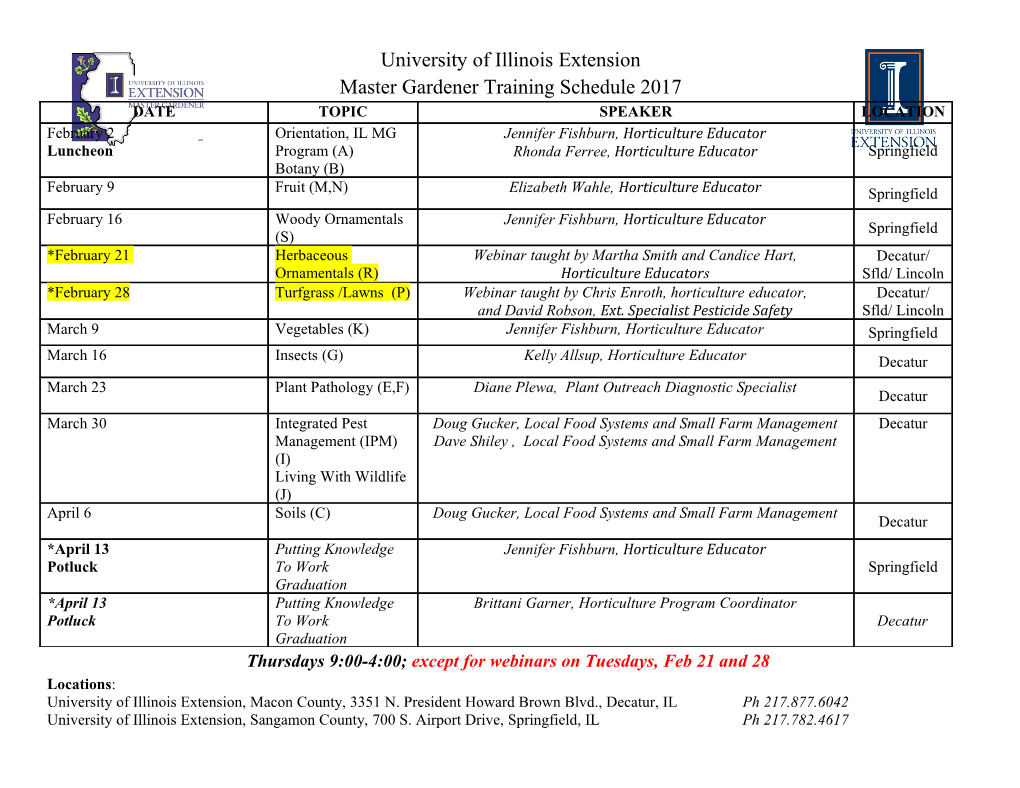
University of Groningen Trans reentrant loop structures in secondary transporters Dobrowolski, Adam Jan IMPORTANT NOTE: You are advised to consult the publisher's version (publisher's PDF) if you wish to cite from it. Please check the document version below. Document Version Publisher's PDF, also known as Version of record Publication date: 2012 Link to publication in University of Groningen/UMCG research database Citation for published version (APA): Dobrowolski, A. J. (2012). Trans reentrant loop structures in secondary transporters. s.n. Copyright Other than for strictly personal use, it is not permitted to download or to forward/distribute the text or part of it without the consent of the author(s) and/or copyright holder(s), unless the work is under an open content license (like Creative Commons). Take-down policy If you believe that this document breaches copyright please contact us providing details, and we will remove access to the work immediately and investigate your claim. Downloaded from the University of Groningen/UMCG research database (Pure): http://www.rug.nl/research/portal. For technical reasons the number of authors shown on this cover page is limited to 10 maximum. Download date: 23-09-2021 Trans reentrant loop structures in secondary transporters Adam J. Dobrowolski Cover design: Adam J. Dobrowolski Cover: The twelve angle stone , in the Hatun Rumiyoc street of Cuzco, Peru. Part of the palace of Inca Roca. Picture taken by author. The work described in this thesis was carried out in the Molecular Microbiology group of the Groningen Biomolecular Sciences and Biotechnology Institute (GBB) of the University of Groningen, The Netherlands, and was financially supported by a grant from the Dutch Organization for Scientific Research (NWO-ALW). ISBN: 978-90-367-5318-0 ISBN: 978-90-367-5319-7 (electronic version) Copyright © 2012 by Adam J. Dobrowolski Printed by: JAKS Wrocław, Poland Printing of this thesis was supported by generous contribution from the University of Groningen and the Groningen Biomolecular Sciences and Biotechnology Institute (GBB). RIJKSUNIVERSITEIT GRONINGEN Trans reentrant loop structures in secondary transporters Proefschrift ter verkrijging van het doctoraat in de Wiskunde en Natuurwetenschappen aan de Rijksuniversiteit Groningen op gezag van de Rector Magnificus, dr. E. Sterken, in het openbaar te verdedigen op maandag 2 april 2012 om 12.45 uur door Adam Jan Dobrowolski geboren op 1 mei 1980 te Zielona Góra, Polen Promotor: Prof. dr. A.J.M. Driessen Copromotor: Dr. J.S. Lolkema Beoordelingscommissie: Prof. dr. K.J. Hellingwerf Prof. dr. B. Poolman Prof. dr. B. Dijkstra Rodzicom Contents Chapter I General introduction. 9 2-domain secondary transport proteins. Chapter II Membrane topology prediction by hydropathy profile alignment: 41 membrane topology of the Na +-glutamate transporter GltS. Chapter III Functional importance of GGXG sequence motifs in putative 59 reentrant loops of 2HCT and ESS transport proteins. Chapter IV Cross-linking of trans reentrant loops in the Na +-citrate 79 transporter CitS of Klebsiella pneumoniae. Chapter V Evolution of antiparallel two-domain membrane proteins. 101 Swapping domains in the glutamate transporter GltS. Chapter VI Summary 119 Chapter VII Samenvatting (summary in Dutch) 127 Chapter VIII Streszczenie (summary in Polish) 135 Abbreviations 143 References 145 List of publications 157 Acknowledgements 158 Chapter I General introduction. 2-domain secondary transport proteins Adam Dobrowolski and Juke S. Lolkema Chapter I Abstract The growing number of reported X-ray crystal structures of membrane proteins has revealed that many of them consist of two homologous domains, or structural repeats, that share a similar fold. This structural motif is especially widespread in the group of secondary transporters. A plausible model for the evolution of two-domain membrane proteins with an internal structural repeat involves a duplication of a primordial gene followed by a fusion, thus resulting in a single gene encoding a protein with two homologous domains. The two domains have the same (parallel) or opposite (antiparallel) orientation in the membrane. The evolution of the antiparallel two-domain membrane proteins requires an ancestral protein with so-called “dual-topology” that inserts into the membrane with random orientation. In this chapter 3D structures of secondary transporters are described with emphasisis on the two-domain structure. Remarkably, secondary transporters that are classified into a large number of different families by sequence similarity (TC system), show a much smaller number of specific folds. 10 General introduction 1. Introduction 2. Structural repeats in membrane proteins 3. Evolution of two-domain membrane proteins 4. Secondary transporters with two domains 4.1. MFS fold (ST[1]) 4.2. LeuT fold (ST[2]) 4.3. CitS/GltS fold (ST[3]) 4.4. DAACS/Glt Ph fold (ST[4]) 4.5. RND fold 4.6. MATE fold 4.7. FNT fold 4.8. ClC fold 4.9. BASS and NhaA fold 4.10. MCF fold 5. Other two-domain membrane proteins 6. Summary 7. Outline of this thesis 11 Chapter I 1. Introduction All living cells are surrounded by biological membranes, which consist of a phospholipid bilayer and a variety of proteins, called membrane proteins. The membrane proteins are responsible for the contact between cell and its environment. Biomembranes are the interface of two compartments, such as the intra- and extracellular environment or mitochondrial matrix and cytosol. Membrane proteins interact closely with both water and lipids in their environment. They are synthesized as other proteins at the ribosome and, at the same time, targeted to the different membrane localization within a cell. Most membrane proteins are involved in transport and signaling, or they are key components in energy transduction, such as converting the chemical energy in ATP into electrochemical energy, or, in reverse, in ATP synthesis. Knowledge about the structure of membrane proteins is an important source of information in molecular biology. Together with functional studies, the structures provide insight into the molecular mechanism by which they work. This knowledge helps in the search for improved medicines, since many targets are membrane proteins responsible for signaling or transport at the cell membrane (69). Recently, the availability of 2D and 3D structures obtained by tools such as electron microscopy (EM) and X-ray crystallography combined with theoretical analysis has significantly increased our understanding of the molecular functionality of membrane proteins. Integral membrane proteins form a considerable part of all proteins in a cell. It was shown that on average about 30% of genes encoded in the genome of a cell code for integral membrane proteins (65, 155). Despite the fact that nowadays almost 300 unique structures of membrane proteins are available in the Protein Data Bank (PDB), this number is still limited in comparison to the many structures published for soluble proteins (6). The low number of crystal structures is related to the difficulties encountered in obtaining crystals that give a good diffraction pattern. Membrane proteins are very hydrophobic and can be only purified in a detergent solubilized state. The hydrophobic surface of the protein provides very few contact points to form a crystal lattice. Nevertheless, in the last decade, great progress has been made in obtaining 3D structures of membrane proteins. In spite of this great progress, it should be noted that transport across membranes, by definition, is a dynamic process and it is difficult to fully 12 General introduction understand the mechanism exclusively on the basis of static pictures, even if they are of high resolution. In spite of a great diversity in functions, integral membrane proteins share a similar architecture. Membrane proteins follow some relatively simple rules governed by their hydrophobic nature and restrictions imposed by the lipid bilayer. Membrane proteins consist of either α-helical bundles or β-barrels (Fig.1). The fully satisfied backbone hydrogen bonding found in these secondary structures avoid unfavorable interactions of backbone amide or carbonyl groups with the hydrophobic environment of the lipid bilayer. With few exceptions, α-helical bundles are found in cytoplasmic and subcellular compartment membranes and β-barrels in the outer membranes of Gram-negative bacteria, mitochondria and chloroplasts (170). The polypeptide chain of membrane proteins composed of a transmembrane α-helical bundle or β-barrel crosses the membrane in a zig-zag fashion. The parts of a chain that connect the transmembrane segments (TMSs) are termed loops. Consequently, a membrane protein contains both intracellular and extracellular loops. Figure 1 . Two types of membrane protein structures. The α-helical (left) and β-barrel (right) membrane proteins. (http://www2.warwick.ac.uk/alumni/services/eportfolios/msrfas/research/) The recent presentation of quite a number of 3D structures of membrane proteins has revealed another feature of membrane proteins that is found in many of them. Many membrane proteins consist of two homologous domains that result in structural repeats and two-fold symmetry in the structure. Structural repeats are found in membrane 13 Chapter I proteins of different functions. They have been observed in channel proteins, the translocon of the protein excretion machinery, complex I of the respiratory chain and are especially frequent in secondary transporters. The structural repeats are
Details
-
File Typepdf
-
Upload Time-
-
Content LanguagesEnglish
-
Upload UserAnonymous/Not logged-in
-
File Pages161 Page
-
File Size-