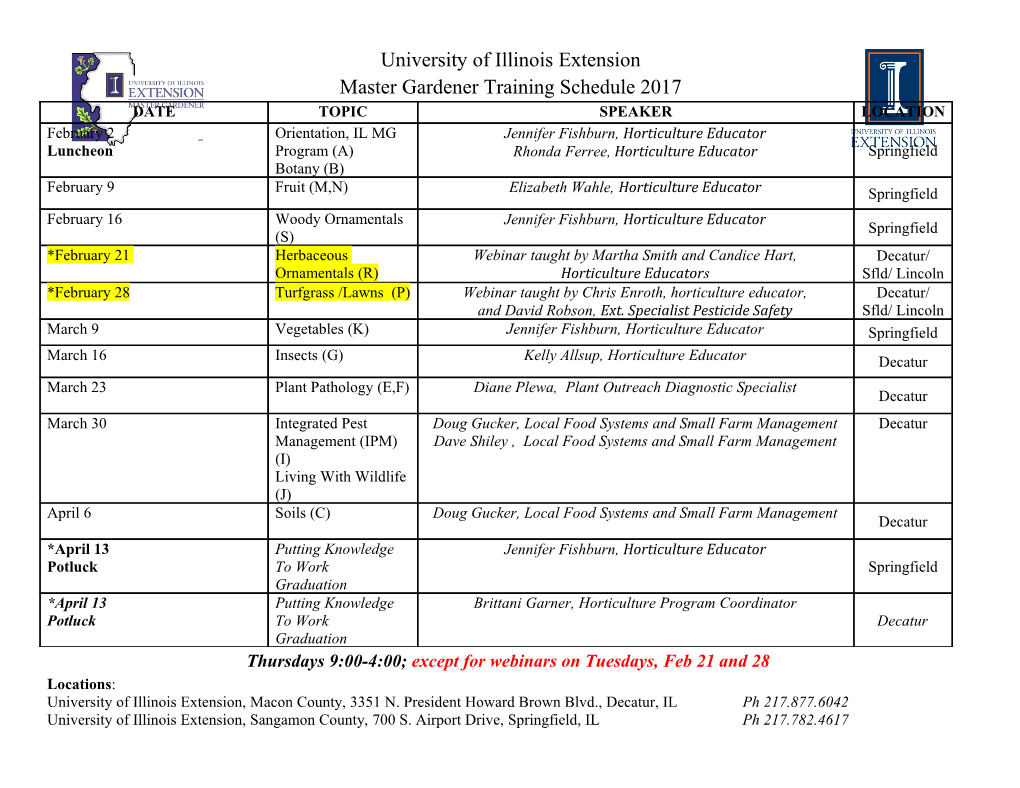
EQUINE ELECTROCARDIOGRAPHY: EXPLORATION OF NEW DIAGNOSTIC STRATEGIES Tinne Verheyen Dissertation submitted in fulfilment of the requirements for the degree of Doctor of Philosophy (PhD) in Veterinary Sciences 2012 Promotors: Prof. dr G. van Loon Prof. dr P. Deprez Prof. dr S.U. Sys Department of Large Animal Internal Medicine Faculty of Veterinary Medicine Ghent University Equine electrocardiography: exploration of new diagnostic strategies Tinne Verheyen Vakgroep Interne geneeskunde en Klinische Biologie van de Grote Huisdieren Faculteit Diergeneeskunde Universiteit Gent ISBN:978-90-5864-313-1 “He who maintains that new knowledge of electrocardiography is no longer possible, ignores history” Dr. Charles Fisch TABLE OF CONTENTS LIST OF ABBREVIATIONS PREFACE 1 GENERAL INTRODUCTION 1. Electrical properties of the heart 7 2. Electrocardiography in human and veterinary medicine 10 CHAPTER 1.1 An overview of electrocardiographic recording techniques in the horse 25 CHAPTER 1.2 An overview of electrocardiographic interpretation in the horse 39 CHAPTER 2 Scientific aims 59 SECTION 1 CHAPTER 3 Electrocardiography in horses with atrial fibrillation 65 CHAPTER 4 Electrocardiography in horses with atypical myopathy 87 SECTION 2 CHAPTER 5 Esophageal electrocardiography in horses 111 CHAPTER 6 Intra-atrial electrocardiography in horses 143 GENERAL DISCUSSION 163 SUMMARY 191 SAMENVATTING 197 CURRICULUM VITAE 205 BIBLIOGRAPHY 209 DANKWOORD 215 LIST OF ABBREVIATIONS 2DST two-dimensional speckle tracking AERP atrial effective refractory period AFCL atrial fibrillation cycle length Am peak radial wall motion velocity during late diastole AM atypical myopathy Ao aortic diameter APD atrial prelature depolarisation AV atrioventricular CD contraction duration by TDI CDSC time to peak circumferential strain CDSL time to peak longitudinal strain CDSR time to peak radial strain CK creatine kinase cTnI cardiac troponin I DHA docosahexaenoic acid E early diastolic filling EAD early afterdepolarisation ECG electrocardiogram E/E10/20/30 intra-esophageal recording with interelectrode distance of 10, 20 or 30 cm Em peak radial wall motion velocity during early diastole E/Shigh/mid/low combined esophageal-surface recording with surface electrode positioned high, mid or low FS fractional shortening HW height at the withers IVRT isovolumic relaxation time IVS interventricular septum MADD multiple acyl-CoA dehydrogenase deficiency mSR maintenance of sinus rhythm LLA left atrial diameter measured from a left parasternal view LAsa left atrial internal diameter measured from a short axis view LVFW left ventricular free wall LVPEP left ventricular pre-ejection period LVET left ventricular ejection time LQTS long QT syndrome MEA mean electrical axis p5 5th percentile P/QRSmagn ratio of P wave magnitude and QRS complex magnitude PSM postsystolic motion rAF recurrence of atrial fibrillation RLA left atrial diameter measured from a right parasternal view QTc QT interval with Fridericia’s correction method ROI region of interest SC peak circumferential strain SD standard deviation SL peak longitudinal strain Sm peak radial wall motion velocity during systole SR peak radial strain STI synchronicity time index TDI tissue Doppler imaging t-MVO time to mitral valve opening T-PMVT torsade-like polymorphic ventricular tachycardia TVEC transvenous electrical cardioversion Uhigh/mid/low unipolar surface recording with electrode positioned high, mid or low VLADD very long-chain acyl-CoA dehydrogenase deficiency VPD ventricular premature depolarization VT ventricular tachycardia PREFACE PREFACE Equine electrocardiography (ECG) is still poorly developed when compared to electrocardiography in human and small animal medicine. Until now, the clinical use of ECG in horses is restricted to surface ECG recordings for diagnosis of rate and rhythm disturbances. Limited attention is given to electrode position and different lead recordings. Apart from a series of studies on vectorcardiography and the clinical significance of changes in T waves fifty years ago, hardly any research was dedicated to the potential of electrocardiography in horses. Nowadays, with affordable wireless ECG units available to the equine clinician, dysrhythmias appear more common than expected. As a result, more questions emerge regarding normal values, diagnosis and mechanisms of cardiac dysrhythmias, driving research into the field of equine dysrhythmias and electrocardiography. In the introduction of this thesis, a brief overview is given of the extensive knowledge regarding cardiac electrograms in human and small animal medicine. In a second part, a summary is provided regarding standard ECG recording in horses with emphasis on the recording technique and the interpretation of the surface ECG. The research part of the thesis is divided in two sections. In the first section the clinical importance of surface ECG recordings was studied in two diseases that are known to affect the heart. The second section describes new recording techniques which have specific advantages to investigate the atrial electrical activity of the equine heart. 3 GENERAL INTRODUCTION GENERAL INTRODUCTION ELECTRICAL PROPERTIES OF THE HEART Throughout history, horses have been admired for their extraordinary athletic ability. Decades of selective breeding have further contributed to the extraordinary cardiovascular capacity of horses. The central and most important organ in maintaining this cardiovascular capacity is the heart. Essentially, the heart functions as a pump. With each contraction, deoxygenated blood transported from the body to the right side of the heart is pumped to the lungs for oxygenation; oxygenated blood returning to the left side of the heart is subsequently distributed throughout the body. In order for this pump function to be effective, the cardiac contraction needs to be completed in an orderly fashion, where first the two atria contract, followed by contraction of both ventricles. Contraction of cardiac myocytes occurs only in response to an action potential. The electrical impulse starts in the sino-atrial (SA) node, located in the right atrium, and spreads sequentially to the left atrium, through the atrioventricular (AV) node, over the Bundle of His, the left and right bundle branches and the terminal fibers of the widely spread Purkinje system to the equine ventricular myocardium.1,2 Upon appropriate stimulation, tiny pores and channels in the cardiac cells open and close in a predefined way to allow specific ions to move across the cell membrane. This movement of ions results in changes in the transmembrane potential and generation of an action potential.3 Each time an action potential is generated, myocardial cells go through a process of depolarization, followed by repolarization and a resting phase. In certain specialized conducting cells, called pacemaker cells, the transmembrane potential does not remain constant in the resting phase, but slowly depolarizes. When this spontaneous depolarization reaches the threshold potential, it generates an action potential by itself, a process called ‘automaticity’ (Fig. 1).3,4 7 GENERAL INTRODUCTION Figure 1: Phases of the cardiac action potential in ventricular myocardial cells (A) and pacemaker cells (B). Phase 0 is the upstroke of the action potential, which is faster in ventricular myocardial cells where it is due to an inward movement of both sodium and calcium. Phase 1 is the rapid repolarization, primarily due to an almost complete inactivation of the inward sodium and calcium current. Phase 2 is the plateau phase of the action potential, which is prominent in ventricular muscle but not in pacemaker cells. It depends on a continued entry of sodium and calcium ions through their major channels and a minor membrane current due to the Na+-Ca2+ exchanger. Phase 3 is the repolarization phase which is due to an outward current of potassium. Phase 4 is the electrical diastolic phase or resting phase of the action potential. In pacemaker cells, changes in the currents of potassium, calcium and the funny current (constituted by sodium and potassium) produce pacemaker activity.5 The rate of spontaneous depolarization is fastest in SA nodal cells, but the AV node and Purkinje fibers also have automaticity and will take over as a pacemaker if the SA node fails to depolarize.5 During the processes of depolarization and repolarization, the movement of ions causes a current to flow across the cell membrane at various points, and a difference in potential arises between one part of the cell and another. When the current is flowing, an electrical field is set up around the cell, which acts as a dipole. When the cell is depolarized or repolarized and hence in a stable state, no current is flowing across the cell, and no electrical field is formed around it. Each myocyte forms its own electrical field, but the individual fields summate to produce an electrical field around the whole heart, which can be regarded as a single dipole.3,6 If the changes in the electrical field are large enough, they can be detected on the body surface by electrocardiography (ECG). The electrical field changes associated with SA node depolarization are too small to be 8 GENERAL INTRODUCTION detected on the surface ECG. But when the electrical impulse spreads from the SA node over the atrial myocardium, the generated electrical potentials produce a P wave on the surface ECG. The impulse is then conducted slowly through the AV node and rapidly over the His bundle and bundle branches, producing electrical
Details
-
File Typepdf
-
Upload Time-
-
Content LanguagesEnglish
-
Upload UserAnonymous/Not logged-in
-
File Pages230 Page
-
File Size-