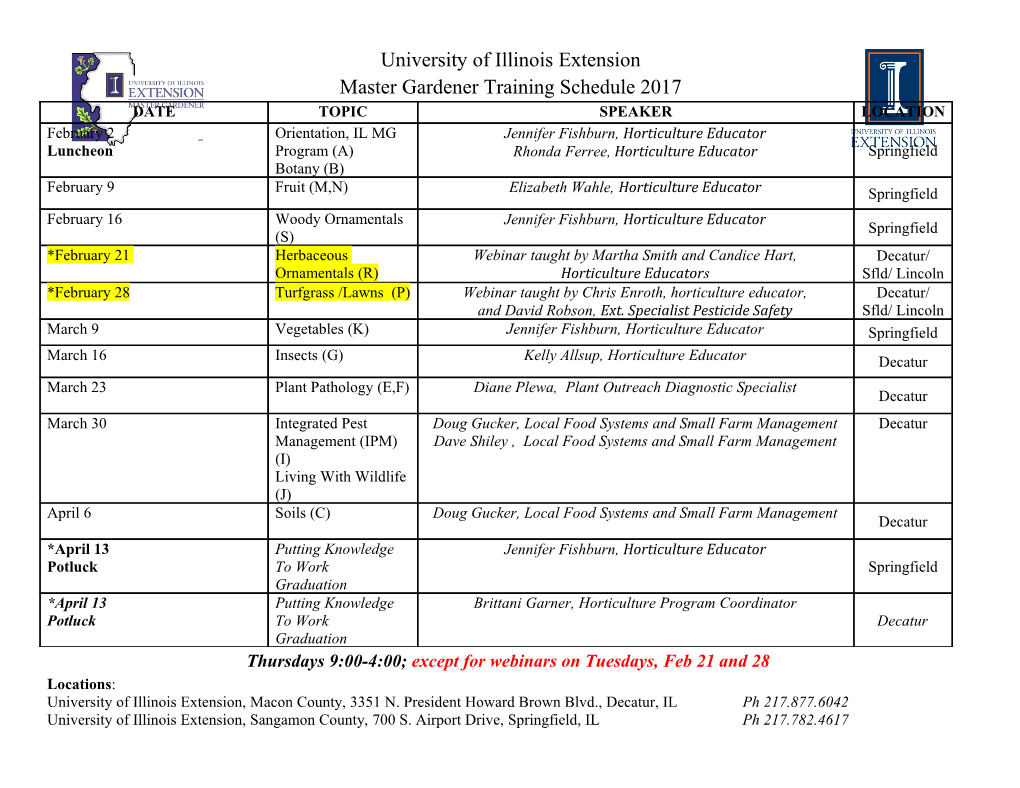
preprint – 1 of 7 The Effects of Freshwater Flows and Salinity on Phytoplankton Biomass and Composition in an Urban Estuary, The Swan River, Western Australia D. P. Hamilton, T. U. Chan, B. J. Robson and B. R. Hodges1 Centre for Water Research, The University of Western Australia, Nedlands, WA 6907, Australia ABSTRACT Estuarine residence times regulate not only biomass, but also phytoplankton succession. In the Swan River estuary, Western Australia, dinoflagellate and marine diatoms tend to dominate during periods of low flow and high residence times, whereas the faster-growing freshwater diatoms and chlorophytes are associated with higher flow rates. Modifications to the estuary and its catchment area over the last century have considerably reduced residence times and may favour dinoflagellate and marine diatom dominated populations. Anecdotal evidence also suggests a greater regularity of summer blooms dominated by these groups in recent years. INTRODUCTION A number of factors make estuaries particularly vulnerable to eutrophication and algal blooms. First, human populations tend to proliferate around estuary margins, often leading to increases in land disturbance and nutrient inputs (Boynton et al., 1992). For example, Australia's geographic demography is associated with high concentrations of population along coastal land margins relative to inland areas and the arid interior in particular. Estuaries are sometimes considered to be the terminal repository for much of the sediment and nutrients derived from terrestrial sources via tributary inflows. They may therefore act to filter sediments and nutrients from inputs to adjoining coastal systems, provided that processes of burial and immobilisation of nutrients are maintained (Fisher et al., 1988). However, density stratification of freshwater and marine water leading to salt wedge formation, a phenomenon associated particularly with estuaries (Geyer, 1989), may lead to oxygen depletion in bottom waters, and subsequent remobilisation of sediment nutrients through reduction processes (Ackroyd et al., 1987). Also, resuspension of bottom material through wind or tidal action may increase water column concentrations of sediments and nutrients (e.g. Mortimer et al., 1998). Well documented cases of stability of buoyancy fronts in the salt wedge region of estuaries, and associated zones of stagnation, may allow sufficient time for net phytoplankton growth to occur before cells are transported seaward and out of the system (Malone et al., 1988; Cloern, 1996). Thus the coalescence of a number of favourable physical and chemical changes in the water column (e.g. light, temperature and nutrients), that allow phytoplankton to grow at near maximal rates, together with sufficient hydraulic residence time in the estuary, will determine whether phytoplankton blooms may occur. However, what is often not fully appreciated is the capacity of estuary residence time to regulate not only the eventual biomass, but also the succession of phytoplankton species. When residence times are of the order of cell doubling times or less, phytoplankton species or taxa that out-compete others by virtue of higher growth rates will be favoured. This effect works in conjunction with the more readily identifiable pattern of phytoplankton succession in estuaries that is associated with salinity gradients, leading to succession amongst freshwater, brackish and marine adapted species (Thompson, 1998). 1 Presently at the Dept of Civil Engineering, University of Texas at Austin, USA. To appear in Proceedings, 3rd International Hydrology and Water Resources Symposium (Hydro 2000), Vol 1, pp. 114- 119, 2000. preprint – 2 of 7 In this paper we examine the relationship of phytoplankton biomass and species composition to tributary inflows and salinity in a Western Australian estuary, the Swan River. We also speculate on how human modifications to the flow regime in the Swan River may have impacted upon bloom frequency, duration and composition of the dominant taxa. STUDY SITE The Swan River estuary is fed by a large catchment (area = 120,000 km2) that is dominated by the Avon River (catchment area = 119,500 km2). Rainfall varies over the catchment from ~ 900 mm yr-1 in coastal regions to ~ 300 mm yr-1 around the eastern boundary, while respective water runoff of these locations per 100 mm of rainfall may be > 70 mm and < 5mm (Viney and Sivapalan, in press). The climate can be classified as Mediterranean, with typically 70% of rainfall confined to the winter and spring months of June to September. Correspondingly, tributary runoff is highly seasonal, with little or no flow occurring in the first 4-5 months of each year in the Avon River. Smaller tributaries, most of which enter on the Swan Coastal Plain, are also highly seasonal, although flow may vary from seasonal (e.g. Ellen Brook) to perennial in the case of some urban drains (Donohue et al., in press). Groundwater inflows, which are mostly associated with sandy soils of the Swan Coastal Plain, vary little seasonally but may contribute up to 10% of freshwater inputs to the estuary in summer and autumn months when flows from surface-fed tributaries are small (Linderfelt and Turner, in press). In summer and autumn, water of marine origin intrudes along the Swan Coastal Plain up the Swan River to approximately 50 km upstream of the estuary mouth at Fremantle (Fig. 1). Winter runoff drives the salt wedge seaward, occasionally close to Fremantle in very wet years (Stephens and Imberger, 1996). Tidal excursions of the salt wedge are typically of the order of 1-3 km although synoptic forcing may displace the salt wedge by around 10 km, corresponding to the duration of passage of low- and high-barometric pressure systems (Hamilton et al. in press). N Ellen Brook Agriculture (1950s-) Perth city Urbanisation (1990s-) Urbanisation Indian Avon River Ocean Clearing, salinisation Fremantle Swan River (1900s-) Channel River training (1958-71) Dredging ~2m to ~14m (1892-) Ascot Waters Helena River Boat harbours Mundaring Canning River and marinas (1990s-) Weir (1902) 1 0 1 2 km Kent Street Weir (1920s) Canning Dam (1940) Figure 1. Map of the Swan River showing major tributaries, estuary mouth, and (in italics) some of the major changes that have had an impact on the estuary hydrology, and the year(s) that the changes occurred. Note the narrow constriction between Canning River and Perth city, which delineates the lower basin towards Fremantle from the upper basin. To appear in Proceedings, 3rd International Hydrology and Water Resources Symposium (Hydro 2000), Vol 1, pp. 114- 119, 2000. preprint – 3 of 7 The highly seasonal hydrology of the Swan River estuary is reflected in a well documented succession of phytoplankton taxa (John, 1994; Thompson and Hosja, 1996). The high flow period of winter and early spring is dominated by freshwater diatoms, which are typically succeeded by a short-lived bloom of freshwater chlorophytes. In summer and autumn, estuarine and marine assemblages are dominant and typically show transitions between dinoflagellates (e.g. Gymnodinium spp. and Prorocentrum spp.) and the cosmopolitan coastal diatom Skeletonema costatum. Blooms of dinoflagellates (Hamilton et al., 1999) and more recently (February, 2000) the blue-green alga Microcystis aeruginosa (Hamilton, 2000) are of particular concern in terms of biodiversity, amenity and long-term impacts on the estuary ecosystem. The hydrology of the Swan River has undergone substantial modifications in the past century (see Fig. 1), and it is likely that these changes have also affected phytoplankton succession. Extensive dredging of the estuary occurred at Fremantle, commencing in 1892, to allow passage of larger boats upriver. The channel entrance at Fremantle has thus been altered from < 2m to ~ 14m, and the main basin below the Narrows (see Fig. 1) is now predominantly marine (Hodgkin and Hesp, 1998). A succession of dams, notably Canning Dam and Mundaring Weir, were constructed for water supply through the 1900s. In their original state these tributaries (i.e. Canning River and Helena River) were unlikely to have exerted a major influence on winter flows, due to the dominance of the Avon River. However, their relative contribution would have been greater in drier months due to the proximity of the tributaries to the high rainfall zone near the coast and the extended period of little or no flow in the Avon River. By contrast, flows in the Avon River are estimated to have increased by 3-4 times over the past 100 years as a result of clearing of remnant vegetation (Viney and Sivapalan, in press). The clearing was particularly widespread between 1940 and 1970. The subsequent increases in runoff prompted adoption of a 'river training scheme', in which large sections of the Avon River were cleared of vegetation ('ripping' of the river bank), then straightened and deepened by bulldozer. It is now generally accepted that this procedure has had a severe impact upon the ecology of these sections of the Avon River and led to major problems with sediment erosion and riverbank stability along many parts of the river (Harris, 1996). Perhaps of even more concern is the rapid increase in salinisation, waterlogging and land degradation in the Avon River catchment, which has resulted from land clearing and reduced water loss(/evapotranspiration) as a result of removal of the remnant vegetation. More recently, rapid growth of the city of Perth has led to the transformation of traditionally rural or natural catchments to urban
Details
-
File Typepdf
-
Upload Time-
-
Content LanguagesEnglish
-
Upload UserAnonymous/Not logged-in
-
File Pages7 Page
-
File Size-