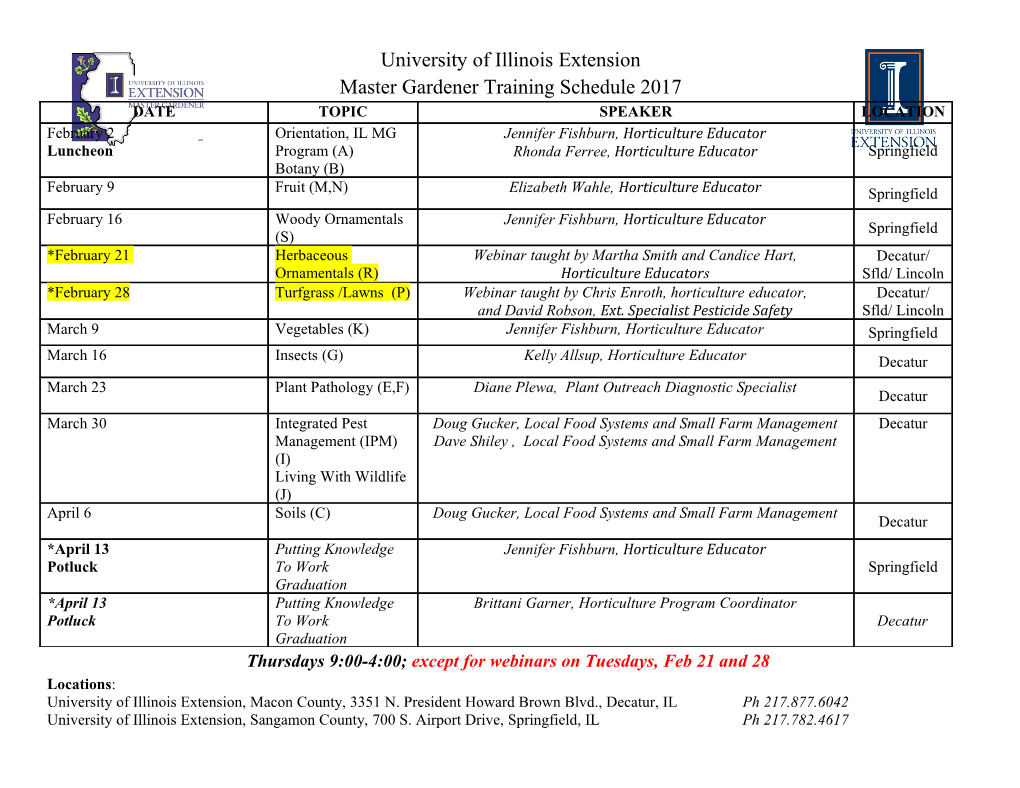
JOURNAL OF GEOPHYSICAL RESEARCH, VOL. 114, B03401, doi:10.1029/2008JB005653, 2009 Volcano load control on dyke propagation and vent distribution: Insights from analogue modeling M. Kervyn,1 G. G. J. Ernst,1 B. van Wyk de Vries,2 L. Mathieu,2,3 and P. Jacobs1 Received 25 February 2008; revised 17 December 2008; accepted 9 January 2009; published 3 March 2009. [1] The spatial distribution of eruptive vents around volcanoes can be complex and evolve as a volcano grows. Observations of vent distribution at contrasting volcanoes, from scoria cones to large shields, show that peripheral eruptive vents concentrate close to the volcano base. We use analogue experiments to explore the control of volcano load on magma ascent and on vent location. Results show that the local loading stress field favors eruption of rising magma away from the volcano summit if a central conduit is not established or is blocked. Two sets of scaled experiments are developed with contrasting rheological properties to analyze similarities and differences in simulated magma rise below a volcano: (1) Golden syrup (magma analogue) is injected into a sand-plaster mixed layer (crust analogue) under a cone; (2) water or air (magma analogues) is injected into gelatin under a sand cone. Rising dykes approaching the cone stress field are stopped by the load compressive stress. With continued intrusion, dyke overpressure builds up; dykes extend laterally until their tips are able to rise vertically again and to erupt in the flank or at the base of the volcano. Lateral offset of the extrusion point relative to the edifice summit depends on substratum thickness, volcano slope, and dyke overpressure. The 3D geometry of Golden syrup intrusions varies with experimental parameters from cylindrical conduits to dyke and sill complexes. Experimental results are compared with illustrative field cases and with previously published numerical models. This comparison enables applications and limitations of the analogue models to be highlighted and allows us to propose a conceptual model for the evolution of vent distribution with volcano growth. Citation: Kervyn, M., G. G. J. Ernst, B. van Wyk de Vries, L. Mathieu, and P. Jacobs (2009), Volcano load control on dyke propagation and vent distribution: Insights from analogue modeling, J. Geophys. Res., 114, B03401, doi:10.1029/2008JB005653. 1. Introduction tures and direction, magma chamber location and size, magma composition [e.g., Bacon,1985;Connor et al., [2] A volcano grows by successive eruptions from a 1992; Fialko and Rubin, 1999; Mazzarini and D’Orazio, central vent and/or from vents on the flanks or around the 2003; Corazzato and Tibaldi, 2006]. Vent distribution in base. It can also grow endogenously by intrusion. Intrusions turn influences volcano growth and morphology. Docu- contribute to edifice construction in a complex way. They menting and identifying factors controlling vent distribution can add volume [Annen et al., 2001], raise slopes, alter load can provide insights into controls on magma plumbing. distribution or cause spreading and collapse. Also, they can [4] It is common to find peripheral vents or to observe deform edifices, changing the stress distribution, and thus eruptions focusing close to a volcano base or at a marked the boundary conditions for future intrusions and ultimately break-in-slope (BIS) on the lower flanks [e.g., Poland et al., eruptions [e.g., Walter and Amelung, 2006]. 2008]. The relationship between topography and vent [3] The distribution of peripheral vents can be complex location can be documented at many volcanoes using and evolve through time. It is controlled by interaction of remote sensing topographic data. However, the process regional and local factors: i.e., regional and local stress leading to preferential vent opening away from the volcano fields, regional structures, volcano shape, spreading struc- summit and close to its base has been little discussed [Shteynberg and Solovyev, 1976; Fialko and Rubin, 1999; Pinel and Jaupart, 2004b; Gaffney and Damjanac, 2006]. 1Mercator and Ortelius Research Centre for Eruption Dynamics, Department of Geology and Soil Sciences, Ghent University, Gent, [5] Numerous dynamic models for dyke (i.e., liquid-filled Belgium. fracture) propagation assuming a homogeneous half-space 2Laboratoire Magma et Volcans, Universite´ Blaise Pascal, Clermont- have been proposed [Johnson, 1970; Pollard, 1973, 1987; Ferrand, France. Dahm, 2000; Menand and Tait, 2002, and references 3Volcanic and Magmatic Processes Research Group, Trinity College Dublin, Dublin, Ireland. therein]. It has been proposed that dyke propagation direc- tion is mainly controlled by regional stress orientation, Copyright 2009 by the American Geophysical Union. presence of planar discontinuities in the host rock, or 0148-0227/09/2008JB005653$09.00 B03401 1of26 B03401 KERVYN ET AL.: VOLCANO LOAD CONTROL ON DYKE PROPAGATION B03401 changes in host rock rheological properties [e.g., Pollard, [Mathieu et al., 2008]. Here the main difference is that the 1973]. Dyke propagation in the crust or within volcanic effect of edifice load is now considered. constructs has also been studied with analogue modeling, [9] To predict propagation and outbreak location before a using liquid injection into gelatin [Pollard, 1973; Hyndman dyke reaches the surface, it is important to understand and Alt, 1987; Menand and Tait, 2002; Kavanagh et al., processes controlling dyke initiation, propagation in the 2006; Rivalta and Dahm, 2006, and references therein]. crust or within a volcanic edifice, and interaction with the Some field studies are available to evaluate results from surrounding rock. The objective of this paper is to illustrate numerical and experimental models [Walker, 1993, 1995, and analyze volcano load control upon dyke ascent in the 1999; Gudmundsson, 2002; Klausen, 2006; Poland et al., upper crust and upon outbreak location. Experiments sim- 2008]. However, the 2D nature of field outcrops limits the ulate dykes ascending from a deep source, below a homog- ability to reconstruct the 3D shape of subvolcanic intru- enous circular cone without any pre-established structure sions. 3D seismic observations has recently shown some (e.g., conduit, rift zone, dyke swarm) controlling magma new insights into the complex shape of intrusive bodies, but propagation. Attention is paid to scaling experiments for with limited success for dykes [e.g., Thomson, 2007]. basalt/andesite magma viscosity, but we expect results to be [6] Some work has been dedicated to the propagation of valid for a wider viscosity range. dykes approaching volcanic constructs. Pinel and Jaupart [2000, 2004b] developed a 2D numerical model predicting 2. Vent Concentration at a Volcano Base that ascending dykes can be blocked underneath high 2.1. Stratocones and Long-Lasting Scoria Cones volcanoes (i.e., cone height 4 km for shields and 2km for stratovolcanoes). Edifice load causes magma storage at [10] The geological evolution and vent distribution at depth, or, if magma is of sufficiently low density, it favors Concepcio´n, Nicaragua, is presented by van Wyk de Vries lateral dyke propagation and extrusion at the volcano base. [1993] and Borgia and van Wyk de Vries [2003]. This Gaffney and Damjanac [2006] numerically modeled effects typical stratovolcano is mostly built through central erup- from topography on a dyke rising under a ridge adjacent to a tions, but about 20 peripheral vents (e.g., scoria cones, tuff lowland. In this model, dykes tend to erupt in lower areas, rings and lava domes) are located between 2.5 and 7 km from mostly because of the geometric effect of topography and, the summit (Figure 1). Peripheral vents to the E and W are to a lesser extent, to the lateral confining stresses from the associated with relatively early stages of volcano growth. ridge. These model predictions have not yet been evaluated They are located on flat terrains, at 200–400 m a.s.l., in experimentally. In this paper, experimental results are pre- association with a circular topographic rise around the sented to evaluate the hypothesis that edifice load affects volcano base, interpreted to be a structure caused by volcano magma ascent as well as vent outbreak spatial distribution. flexure. Other peripheral vents are located on the lower flanks [7] Previous gelatin models have documented that stress (i.e., slope <15°) along a N–S structure related to more field reorientation from surface loading (i.e., presence of a recent volcano spreading [Borgia and van Wyk de Vries, volcano) causes focusing of ascending dykes below the load 2003]. axial zone [Dahm, 2000; Muller et al., 2001; Watanabe et [11] The base of steep flanks is identified as a preferential al., 2002]. Using injection of hot gelatin into a gelatin block location for vents at other steep conical volcanoes; e.g., at overlain by a gelatin cone, Hyndman and Alt [1987] Arenal, Costa Rica [Borgia et al., 1988], and Mount Adams, observed that as dykes approached the volcano base, they USA [Hildreth and Fierstein, 1997]. Seven of the ten extended laterally, although this process was not fully Holocene vents at Mount Adams are located within 2 km documented or discussed. Within cones, dykes have also below the break-in-slope (BIS) between the steep rubbly been observed to reorientate locally perpendicular to topo- cone and less steep flank lava apron. At Arenal and Mount graphic contours [McGuire and Pullen, 1989] and to reori- Adams, BIS vent concentration is attributed to a lithological
Details
-
File Typepdf
-
Upload Time-
-
Content LanguagesEnglish
-
Upload UserAnonymous/Not logged-in
-
File Pages26 Page
-
File Size-