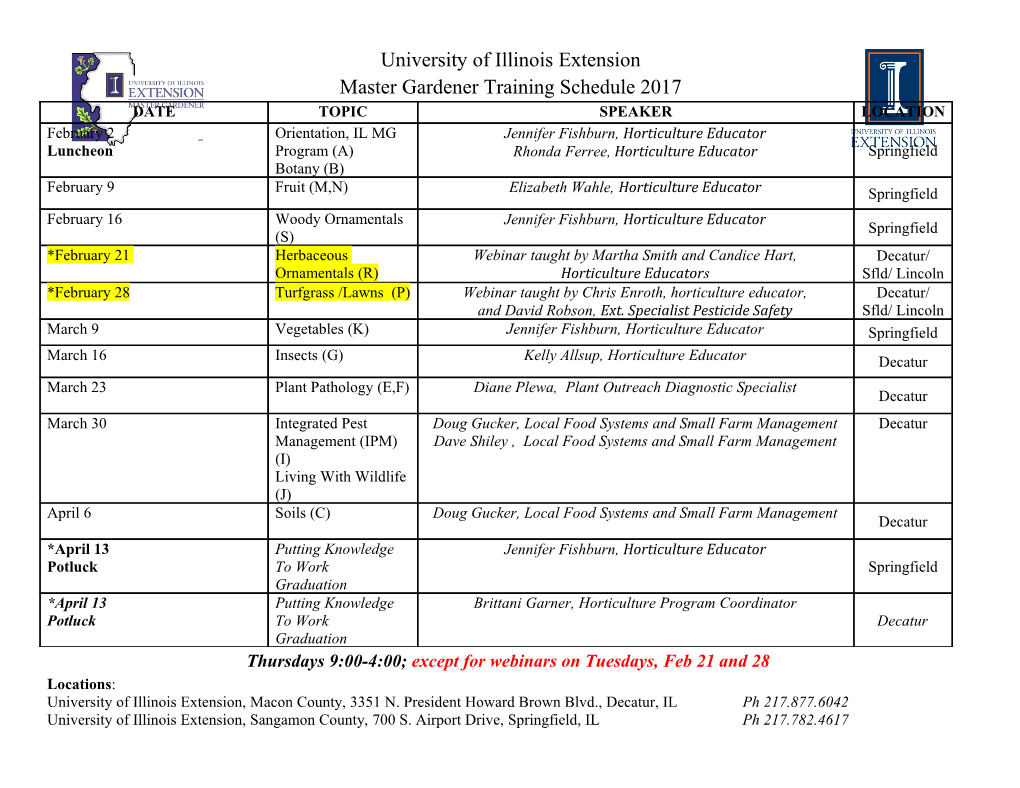
Formation of a symbiotic host-microbe interface: the role of SNARE-mediated regulation of exocytosis of SNARE-mediated regulation the role interface: host-microbe Formation of a symbiotic FORMATION OF A SYMBIOTIC HOST-MICROBE INTERFACE: THE ROLE OF SNARE-MEDIATED REGULATION OF Rik Huisman 2018 Rik EXOCYTOSIS Rik HUISMAN Formation of a symbiotic host-microbe interface: the role of SNARE-mediated regulation of exocytosis Rik Huisman Thesis commitee Promotor Prof. Dr A.H.J. Bisseling Professor of Molecular Biology Wageningen University & Research Co-promotor Dr E.H.M. Limpens Assistant professor, Laboratorry of Molecular Biology Wageningen University & Research Other members Prof. Dr D. Weijers, Wageningen University & Research Prof. Dr C. Gutjahr, LMU, Munich, Germany Dr M.A.C.J. Kwaaitaal, University of Amsterdam Dr M.J. Ketelaar, Wageningen University & Research This research was conducted under the auspices of the Graduate School Experimental Plant Sciences Formation of a symbiotic host-microbe interface: the role of SNARE-mediated regulation of exocytosis Rik Huisman Thesis submitted in fulfilment of the requirements for the degree of doctor at Wageningen university by the authority of the Rector Magnificus Prof. Dr A.P.J. Mol, in the presence of the Thesis Committee appointed by the Academic Board to be defended in public on Friday 16 February 2018 at 4 p.m. in the Aula. Rik Huisman Formation of a symbiotic host-microbe interface: the role of SNARE-mediated regulation of exocytosis 158 pages. PhD thesis, Wageningen University, Wageningen, the Netherlands (2018) With references, with summary in English ISBN: 978-94-6332-317-8 DOI: https://doi.org/10.18174/432066 Table of Contents Chapter 1 7 General Introduction Chapter 2 21 Haustorium formation in Medicago truncatula roots infected by Phytophthora palmivora does not involve the common endosymbiotic program shared by AM fungi and rhizobia Chapter 3 43 A symbiosis-dedicated SYNTAXIN OF PLANTS 13II isoform controls the formation of a stable host-microbe interface in symbiosis Chapter 4 75 Functional redundancy among plant exocytotic SNARE proteins to form a symbiotic interface Chapter 5 99 Specialization of SYP132α to control arbuscule functionality Chapter 6 117 General Discussion Summary 133 References 137 List of publications 152 Acknowledgements 153 Education statement 156 Chapter 1 General Introduction Chapter 1 Plants rely heavily on symbiosis with micro-organisms in the soil to increase their access to scarce nutrients. Especially nitrogen and phosphorous are elements that are growth limiting for plants in most ecosystems (Harpole et al., 2011). Symbiosis is an integral aspect of plant nutrition, as perhaps the only place were plants grow without microbes is the lab of a biologist. Symbiosis classically refers to any relationship of organisms living together, regardless of the resulting benefits or costs this brings for both partners. However, in this 1 thesis I will use the term symbiosis to describe interactions of plants with microbes that are on average mutual beneficial for both partners. A common example is the interaction of plants with bacteria that are free-living in the soil that release nutrients from insoluble or organic compounds that remain otherwise inaccessible to the plant (Clarholm, 1985; Becquer et al., 2014). Plants actively promote these bacteria by secretion of mucilage containing among others carbohydrates and amino acids. Nevertheless, the commitment of both partners is relatively low since there is competition for compounds secreted by the plant and the released nutrients between all soil inhabitants. Endosymbiosis is a far more advanced and intimate form of symbiosis. During endosymbiosis, all or part of the microbe is hosted within the plant cell, which allows a targeted exchange of carbon and nutrients between the two partners. The most widespread and ancient form of endosymbiosis is the interaction of plants with arbuscular mycorrhizal (AM) fungi, which form an extension to the plant root system and help plant to take up nutrients. Many (if not all) other endosymbi- oses that evolved later make use of mechanisms and plant genes involved in AM symbiosis. A key example of this are nitrogen fixing rhizobia that interact with leguminous plants. Most (if not all) microbes that enter living plant cells remain surrounded by a plant derived membrane. AM fungi form arbuscules in plant cells. Arbuscules are highly branched feeding structures that are surrounded by the peri-arbuscular membrane. Rhizobia are completely taken up in plant cells, forming (transient) N2-fixing organelles called symbiosomes, which are surrounded by the so-called peri-bacteroid membrane. These peri-microbial membranes are the main site of nutrient exchange between both partners and their protein composition is highly specialized for this role. This places the peri-micro- bial membranes at the heart of endosymbiosis as they establish an intimate host-microbe interface. Understanding the formation and maintenance of the peri-microbial membrane is therefore crucial to understand endosymbiosis. Recent studies have revealed that the formation of the peri-arbuscular membrane and the peri-bacteroid membrane depend on at least partially overlapping sets of plant genes (Huisman et al., 2012). Besides mutualist symbionts, also (hemi-)biotrophic filamentous pathogens infect living plant cells in which they are surrounded by a peri-microbial membrane. If symbiotic and pathogenic peri-mi- crobial membrane formation depend on similar mechanisms, then understanding the mechanisms that guide accommodation of symbionts will also help understanding the mechanisms that guide accommodation of pathogens. In this thesis, I will study how plant membrane trafficking is regulated to create a symbiotic host-microbe interface. Further, I will study whether the formation of a symbiotic and a pathogenic host-microbe interface depend on the same plant genes. 8 General Introduction Arbuscules and arbuscular mycorrhiza symbiosis Most plants can engage in a symbiosis with filamentous fungi that transfer nutrients to the plant, in exchange for carbohydrates produced by the pant. This means that the fungi effectively form an extension to the plant root system, increasing the total volume of soil from which nutrients can be taken up. Mycorrhiza especially increase the uptake of nutrients that are limited by their low solubility and low diffusion in the soil, like phospho- rous and zinc (Mosse, 1973; Smith & Read, 2008). These nutrients are quickly depleted in 1 the soil directly surrounding the plant root (Lewis & Quirk, 1967). Mycorrhizal fungi extend beyond this zone and are less prone to create depletion zones themselves due to their small diameter. Mycorrhizal fungi also transfer more mobile nutrients like nitrogen to the plant (Leigh et al., 2009), but the importance of mycorrhiza for nitrogen nutrition seems to be limited (Smith & Read, 2008). Besides increasing access to nutrients, mycorrhiza can enhance the uptake of water (Augé, 2001), or increase plant resistance to biotic stresses (Pozo et al., 2010). There are different forms of mycorrhizal symbiosis: Ectomycorrhizal fungi form a symbiosis with many temperate forest trees. Ectomycorrhizal fungi do generally not enter living plant cells, but form a network of hyphae called ‘Hartig net’ that surrounds epidermal cells and which forms the host-microbe interface. The fungal partners that can form ectomyc- orrhiza are diverse (more than 20.000 species), as ectomycorrhiza evolved approximately 60 independent times from a wide range of saprotrophic ancestors (Martin et al., 2016). Endomycorrhizal fungi infect living plant cells. Some specific endomycorrhizal relations are formed by ericoid mycorrhiza and orchid mycorrhiza that are both restricted to inter- action with a single plant family. Arbuscular mycorrhiza (AM) are the most ancient and widespread form of mycorrhizal symbiosis. AM fungi are all part of the phylum Glomer- omycota, and as obligate biotrophs they fully depend on plants for their carbon supply. AM symbiosis dates back 450-460 million years, to the first plants that colonized land (Redecker et al., 2000; Wang et al., 2010b). Today, around 80% of all land plants is able to form a symbiosis with AM fungi. The infection of plants by AM fungi starts with the formation of a hyphopodium on the plant root, from which a hypha emerges that penetrates an epidermal cell. The growth of hyphae through cells requires the active contribution of the plant. Preceding the fungal invasion, a pre-penetration apparatus is formed that consists of an ER- and cytoskel- eton-rich cytoplasmic column that predicts the path of the invading fungus (Genre et al., 2005). After passing the epidermis, the fungus colonizes the root cortex by forming either inter- or intracellular hyphae. When the fungus reaches the inner cortical cell layers, arbuscules are formed. The plant cells harboring an arbuscule, as well as the arbuscules themselves are optimized for the exchange of carbon and nutrients: The membrane contains symbiosis dedicated phosphate transporters (Harrison et al., 2002; Yang et al., 2012; Breuillin-Sessoms et al., 2015), Ammonium transporters (Guether et al., 2009; Kobae 8 9 Chapter 1 et al., 2010), lipid transporters (Zhang et al., 2010a), and proton pumps that create a elec- trochemical potential across the membrane that energizes the transporters (Krajinski et al., 2014; Wang et al., 2014). The arbuscular cells increase their production of fatty acids,
Details
-
File Typepdf
-
Upload Time-
-
Content LanguagesEnglish
-
Upload UserAnonymous/Not logged-in
-
File Pages160 Page
-
File Size-