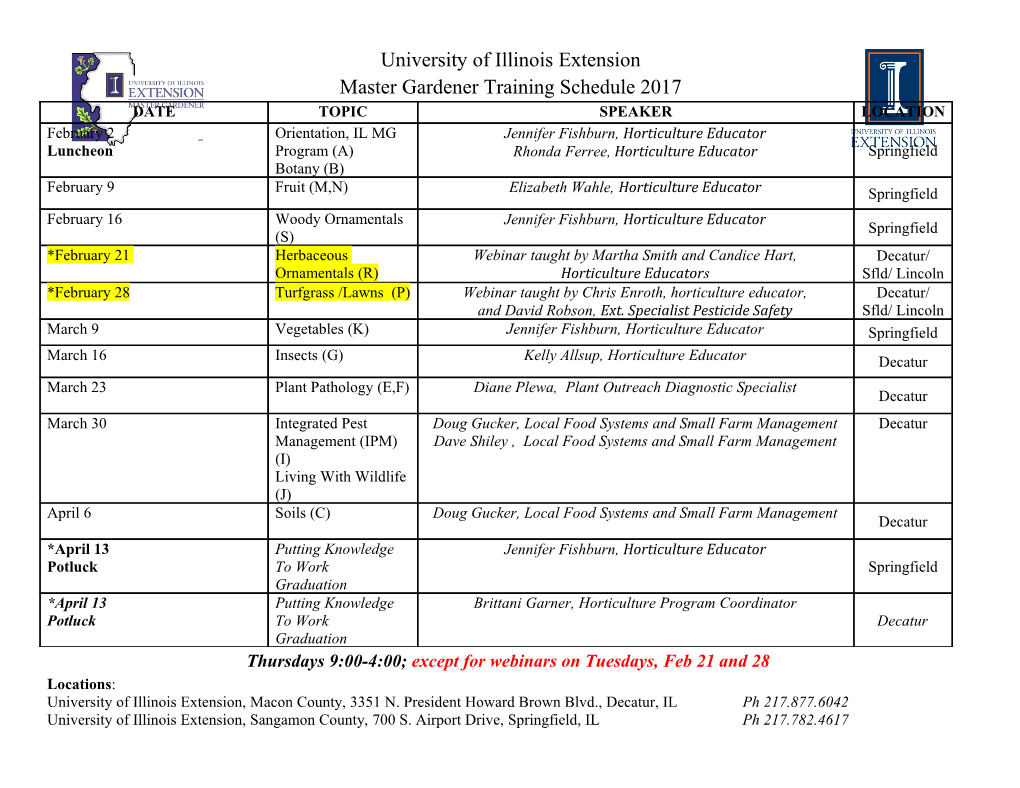
Downloaded from genesdev.cshlp.org on December 3, 2010 - Published by Cold Spring Harbor Laboratory Press Running hot and cold: behavioral strategies, neural circuits, and the molecular machinery for thermotaxis in C. elegans and Drosophila Paul A. Garrity, Miriam B. Goodman, Aravinthan D. Samuel, et al. Genes Dev. 2010 24: 2365-2382 Access the most recent version at doi:10.1101/gad.1953710 References This article cites 142 articles, 52 of which can be accessed free at: http://genesdev.cshlp.org/content/24/21/2365.full.html#ref-list-1 Email alerting Receive free email alerts when new articles cite this article - sign up in the box at the service top right corner of the article or click here Topic Articles on similar topics can be found in the following collections Collections Neurobiology (41 articles) To subscribe to Genes & Development go to: http://genesdev.cshlp.org/subscriptions Copyright © 2010 by Cold Spring Harbor Laboratory Press Downloaded from genesdev.cshlp.org on December 3, 2010 - Published by Cold Spring Harbor Laboratory Press REVIEW Running hot and cold: behavioral strategies, neural circuits, and the molecular machinery for thermotaxis in C. elegans and Drosophila Paul A. Garrity,1,2,5 Miriam B. Goodman,3 Aravinthan D. Samuel,4 and Piali Sengupta1,2,6 1Department of Biology, Brandeis University, Waltham, Massachusetts 02454, USA; 2National Center for Behavioral Genomics, Brandeis University, Waltham, Massachusetts 02454, USA; 3Department of Molecular and Cellular Physiology, Stanford University, Stanford, California 94305, USA; 4Department of Physics and Center for Brain Science, Harvard University, Cambridge, Massachusetts 02138, USA Like other ectotherms, the roundworm Caenorhabditis guided behaviors that operate within each animal’s nor- elegans and the fruit fly Drosophila melanogaster rely on mal thermal zone. behavioral strategies to stabilize their body temperature. Both animals use specialized sensory neurons to detect A note on terminology small changes in temperature, and the activity of these thermosensors governs the neural circuits that control Various terms have been used to describe patterns of migration and accumulation at preferred temperatures. thermotactic movement in C. elegans and Drosophila. Despite these similarities, the underlying molecular, For instance, worms moving down temperature gradients neuronal, and computational mechanisms responsible toward cooler temperatures are said to be ‘‘cryophilic’’ for thermotaxis are distinct in these organisms. Here, (Fig. 1A), while the same behavior executed by flies is we discuss the role of thermosensation in the develop- called ‘‘warmth avoidance.’’ By the same logic, move- ment and survival of C. elegans and Drosophila,and ments up temperature gradients have been called ‘‘ther- review the behavioral strategies, neuronal circuits, and mophilic’’ in worms and ‘‘cold avoidance’’ behavior in molecular networks responsible for thermotaxis behavior. flies (Fig. 1B). Here, movement down and up temperature gradients will be referred to as ‘‘negative thermotaxis’’ and ‘‘positive thermotaxis,’’ respectively. In addition to The ability to sense and respond to ambient temperature positive and negative thermotaxis, worms have a robust is crucial for the survival and fitness of all animals. tendency to move along isothermal contours when they Endotherms such as birds and mammals maintain a rela- are near their preferred temperature, a behavior referred tively constant body temperature regardless of ambient to as ‘‘isothermal tracking’’ (Fig. 1E). Isothermal tracking temperature via a number of mechanisms, including has not been observed in flies. behavioral modification, as well as central regulation of autonomic nervous system functions (Hensel 1973; Simon et al. 1986). However, for ectotherms like Caeno- The ethology of thermotaxis in C. elegans rhabditis elegans and Drosophila melanogaster, whose and D. melanogaster body temperature varies with ambient temperature, be- Temperature is an environmental variable that affects the havioral strategies are the primary mechanism for regu- rate and nature of all chemical reactions, and hence has lating optimal internal temperature (Stevenson 1985; dramatic effects on animal physiology. At the extremes of Huey et al. 2003). In this review, we describe the known the temperature spectrum, exposure to excessive heat or neuronal and molecular strategies used by C. elegans and cold causes dramatic perturbations in cellular physiology Drosophila to detect and behaviorally respond to changes that rapidly lead to the failure of nervous system function in temperature, focusing particularly on temperature- and to serious tissue damage. Thus, thermal nocicep- tion—the ability to sense and respond to noxious heat and noxious cold—is critical for animal survival (Tominaga and Caterina 2004). [Keywords: C. elegans; Drosophila; TRP; cGMP; thermosensory; thermotaxis] Even at more moderate temperatures, thermal variations 5Authors are listed alphabetically. affect animal physiology. Thus, thermotaxis—the ability to 6Corresponding author. E-MAIL [email protected]; FAX (781) 736-3107. migrate up or down a temperature gradient—also makes Article is online at http://www.genesdev.org/cgi/doi/10.1101/gad.1953710. a critical contribution to an animal’s fitness. Thermotaxis GENES & DEVELOPMENT 24:2365–2382 Ó 2010 by Cold Spring Harbor Laboratory Press ISSN 0890-9369/10; www.genesdev.org 2365 Downloaded from genesdev.cshlp.org on December 3, 2010 - Published by Cold Spring Harbor Laboratory Press Garrity et al. Figure 1. Stochasticity, determinism, and decision- making in C. elegans and Drosophila thermotaxis. (A,B) Biased random walks. Trajectories of individual C. elegans nematodes or D. melanogaster larvae are intrinsically stochastic, resembling biased random walks when viewed at low magnification. Each trajec- tory is marked by periods of forward movement (runs) interrupted by abrupt reorientation maneuvers (turns). (A) Trajectories of four worms grown at 15°C and placed on a temperature gradient above Tc. All worms exhibit negative thermotaxis, but no two trajectories are alike. (B) Trajectories of four Drosophila larvae exhibiting positive thermotaxis moving from below preferred tem- perature toward warmer temperatures. (Gray circles) Starting points. (C) Unbiased reorientation in C. ele- gans. The initial run, in which the worm is headed to the lower left, is terminated by a reversal. The reversal is terminated by an V turn. Following the V turn, the worm starts a new run in a new direction. The worm biases its random walk by regulating the frequency of these abrupt turns, depending on direction with respect to the surrounding temperature gradient. Runs are lengthened toward preferred temperatures and short- ened away from preferred temperatures. (D) Biased reorientation in D. melanogaster. During each reorien- tation maneuver, a Drosophila larva will pause and deliberately swing its head back and forth. If the larva happens to be isothermally aligned, it will encounter falling temperatures if it moves its head in one direction (illustrated by blue dots that trace the trajectory of the thermosensory neurons at the larva’s head) and rising temperatures if it moves its head in the other direction (the trace of red dots). The larva biases the probability of starting a new run based on the temperature it encoun- ters during each head sweep. Thus, after being isother- mally aligned, the larva tends to start new runs toward the larva’s preferred temperature. (E) C. elegans steers in a deterministic manner to maintain isothermal alignment when near its Tc (isothermal tracking). During isothermal tracking, C. elegans continuously swings its head back and forth; thus, the thermosensory neurons at its head encounter regular and alternating phases of falling temperature (blue dots) and rising temperature (red dots). The worm uses this sinusoidal variation in temperature to correct the curvature of its undulation, veering its nose away from every temperature variation and stabilizing isothermal alignment to within ;0.01°C. (F) When C. elegans is exposed to positive temperature pulses when it swings its head to one side (filled red dots) but not the other side (open red dots), it curves its overall trajectory away from the thermal stimulation. (G) Comparison of behavioral strategies that govern C. elegans thermotaxis, D. melagnoster larval thermotaxis, and Escherichia coli chemotaxis. is especially important in small ectotherms such as C. their most internal structures in response to changes in elegans and Drosophila, whose small mass (e.g., adult flies surface temperature is less than a tenth of a second. Even are <2 mg) means they have small heat capacities. Thus, D. melanogaster third instar larvae and adult flies are only internal structures readily equilibrate to the temperature of 1–1.25 mm thick, leading to a predicted equilibration of their surroundings (Stevenson 1985; Heinrich 1993). When their most internal structures within a few seconds. Such considering how rapidly this occurs, it is important to rapid equilibration with their environment suggests that consider the distances over which thermal energy must behavioral strategies for seeking out appropriate environ- travel to reach internal structures. The time scale of the ments are paramount for body temperature control in equilibration of internal structures in response to changes these organisms. Moreover, this suggests that, in contrast in surface temperature can
Details
-
File Typepdf
-
Upload Time-
-
Content LanguagesEnglish
-
Upload UserAnonymous/Not logged-in
-
File Pages19 Page
-
File Size-