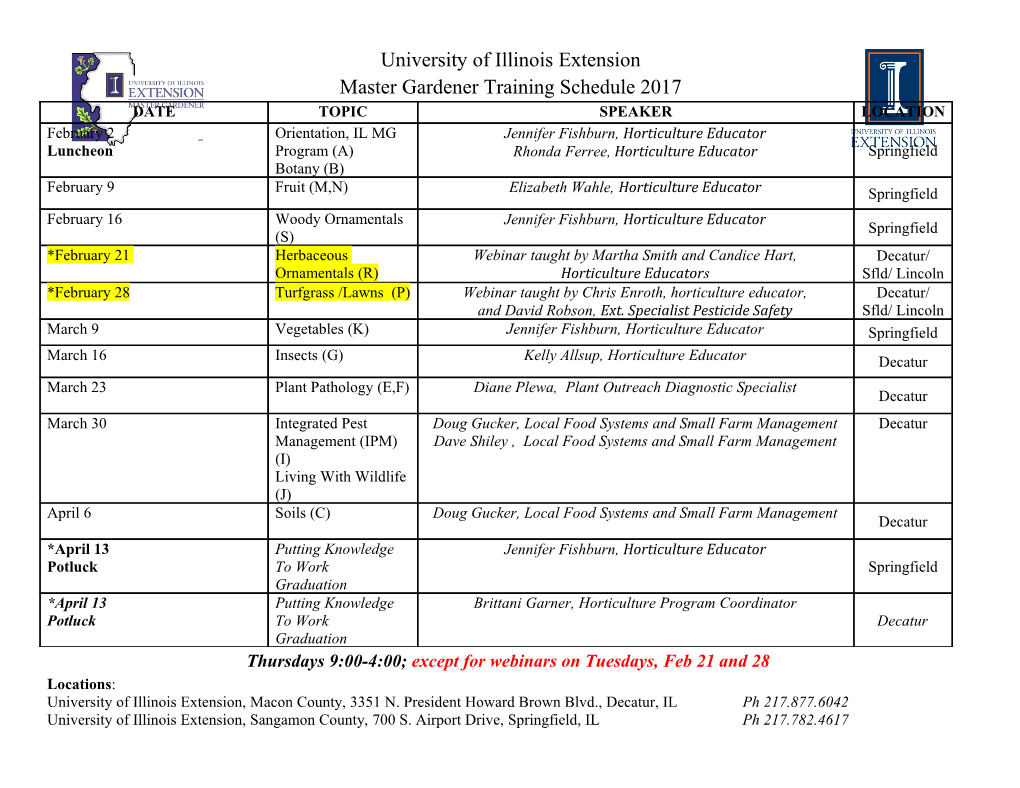
Subscriber access provided by FRITZ HABER INST DER MPI Article High-Throughput Investigation of the Geometry and Electronic Structures of Gas-Phase and Crystalline Polycyclic Aromatic Hydrocarbons Bohdan Schatschneider, Stephen Monaco, Jian-Jie Liang, and Alexandre Tkatchenko J. Phys. Chem. C, Just Accepted Manuscript • DOI: 10.1021/jp5064462 • Publication Date (Web): 07 Aug 2014 Downloaded from http://pubs.acs.org on August 12, 2014 Just Accepted “Just Accepted” manuscripts have been peer-reviewed and accepted for publication. They are posted online prior to technical editing, formatting for publication and author proofing. The American Chemical Society provides “Just Accepted” as a free service to the research community to expedite the dissemination of scientific material as soon as possible after acceptance. “Just Accepted” manuscripts appear in full in PDF format accompanied by an HTML abstract. “Just Accepted” manuscripts have been fully peer reviewed, but should not be considered the official version of record. They are accessible to all readers and citable by the Digital Object Identifier (DOI®). “Just Accepted” is an optional service offered to authors. Therefore, the “Just Accepted” Web site may not include all articles that will be published in the journal. After a manuscript is technically edited and formatted, it will be removed from the “Just Accepted” Web site and published as an ASAP article. Note that technical editing may introduce minor changes to the manuscript text and/or graphics which could affect content, and all legal disclaimers and ethical guidelines that apply to the journal pertain. ACS cannot be held responsible for errors or consequences arising from the use of information contained in these “Just Accepted” manuscripts. The Journal of Physical Chemistry C is published by the American Chemical Society. 1155 Sixteenth Street N.W., Washington, DC 20036 Published by American Chemical Society. Copyright © American Chemical Society. However, no copyright claim is made to original U.S. Government works, or works produced by employees of any Commonwealth realm Crown government in the course of their duties. Page 1 of 36 The Journal of Physical Chemistry 1 2 3 High-Throughput Investigation of the Geometry and Electronic Structures of Gas-Phase 4 5 and Crystalline Polycyclic Aromatic Hydrocarbons 6 7 Bohdan Schatschneider a*, Stephen Monaco a, Jian-Jie Liang b, and Alexandre Tkatchenko c 8 9 a The Pennsylvania State University, Fayette-The Eberly Campus 10 b 11 Accelrys Inc., 5005 Wateridge Vista Drive, San Diego, CA 92121 USA c 12 Fritz-Haber-Institut der Max-Planck-Gesellschaft, Faradayweg 4-6, D-14195 Berlin, Germany 13 14 15 Abstract : The quest for cheap, light, flexible materials for use in electronic applications has 16 resulted in the exploration of soft organic materials as possible candidates, and several polycyclic 17 18 aromatic hydrocarbons (PAH) have been shown to be versatile (semi) conductors. In this 19 20 investigation, dispersion inclusive density functional theory is used to explore all of the current 21 22 crystalline PAHs within the Cambridge Structure Database (CSD) from both structural and 23 24 electronic standpoints. Agreement is achieved between the experimental and calculated 25 crystalline structures as well as the electronic properties: Specifically, variation between the 26 27 mass densities, unit cell parameters and intermolecular close contact fractions were within +5%, 28 29 ±2%, and ±1% of experiment, respectively. It is found that a simple addition of a ~1 eV constant 30 31 to the DFT-PBE gaps provides good agreement with the experimental optical gaps of both gas 32 phase (within ±2.6%) and crystalline (within ±3.5%) PAHs. Structural and electronic analysis 33 34 revealed several correlations/trends; where ultimately, limits in the band gaps as a function of 35 36 structure are established. Finally, analysis of the difference between band gaps of the isolated 37 /'ͯ*' /'ͯ*' 38 molecules and crystals ( Ϛ̿ " ) demonstrates that Ϛ̿ " can be captured qualitatively 39 40 by PBE and PBE0 functionals, yet significant quantitative deviations remain between these 41 functionals and experiment. 42 43 44 45 46 47 48 Keywords : Dispersion Corrected Density Functional Theory (DFT-D), Organic Molecular 49 Crystals, Band Gap, Organic Semiconductors. 50 51 52 53 54 *Corresponding Author: Email: [email protected] Phone: 1 (724) 430-4257 55 56 57 58 59 60 ACS Paragon Plus Environment The Journal of Physical Chemistry Page 2 of 36 1 2 3 1. Introduction : The quest for inexpensive, flexible, light weight materials for use in 4 5 electronic applications has led researchers to explore what useful arrays can be constructed 6 7 through organic means, and the ability to predict useful properties within organic-electronic 8 9 materials is quickly becoming an essential part of organo-electronic product design. Polycyclic 10 11 aromatic hydrocarbons (PAH) make up a group of organic molecular crystals (OMC) which have 12 shown promise for use in electronics and electro-optics 1, 2 . Despite their versatility and 13 14 abundance, theoretical exploration of the electronic properties of most crystalline PAHs remains 15 16 uncharted. Here we use dispersion inclusive density functional theory (DFT) to shed light onto 17 18 the structural and electronic trends occurring within this promising group of materials. 19 The topology of individual PAH molecules can be classified as: 1) linear/nanoribbons 20 21 (comprised of homologous groups of oligoacenes, phenacenes, and oligorylenes) 2) circular 22 23 flakes/discs (K-region PAHs and circumacenes) and 3) triangular. Within each topology two 24 25 edge/periphery types exist: 1) zig-zag and 2) arm-chair. In general, it is known that in a given 26 topology of PAHs (as well as nanoflakes) that the size of the band gap (E ) decreases 3-5 as the 27 g 28 number of aromatic rings (carbon atoms) increases, where arm-chair edge PAHs have larger 29 30 band gaps and cohesive energies (enthalpies of formation) than their zig-zag counter parts. 31 5 32 Similar trends can also be found in fully benzenoid PAHs . It is then understood that the 33 34 ionization energy (I) decreases and the electron affinity (A) increases with increasing number of 35 rings in a homologous class 4 (this follows from the above E -trend as the fundamental/transport 36 g 6 37 gap is I-A) . It is also recognized that as the number of edge atoms (N s) increase with respect to 38 3 39 the total number of atoms (N), the cohesive energy decreases . 40 41 Traditionally, PAHs can be either heterocyclic or only carbon containing, and assemble 42 in molecular crystalline arrays under ambient conditions. Those PAHs containing only hydrogen 43 44 and aromatic carbon can be classified into five crystalline motifs, definable by the π⁰-parameter 45 46 (a product of the inter-planar angle between molecular components and the fraction of C···C 47 7 48 intermolecular close contacts ). The five motifs: a) herringbone (HB) b) sandwich-herringbone 49 50 (SHB) c) beta-herringbone (β-HB) d) gamma (γ) and e) beta (β) are depicted in Figures 1a-1e 51 using structures which exemplify the characteristics of each motif 8,9. 52 53 Each motif has characteristic amounts of C···C (π···π), C···H, and H···H intermolecular 54 55 close contact interactions7-10 . It is known that the HB and β-HB structures are heavily dependent 56 57 on C···H close contact interactions for crystal stabilization as calculated via Hirshfeld surface 58 59 60 ACS Paragon Plus Environment Page 3 of 36 The Journal of Physical Chemistry 1 2 3 analysis 7, but get the least amount of crystal stabilization from C···C close contacts on average 4 5 when compared to other motifs. The SHB structures are dependent on C···H interactions as 6 7 well, but become increasingly dependent on C···C interactions compared to the HB and β-HB 8 9 motifs as half of each molecular component is involved in π···π stacking interactions. The β and 10 11 γ motifs are the most dependent on C···C interactions as the large molecular components have 12 low Ns/N ratios (compared to other motifs), providing ample π-orbitals for stacking interactions. 13 14 15 16 17 18 19 20 21 22 23 24 (c) 25 (a) (b) 26 27 28 29 30 31 32 33 (e) 34 (d) 35 36 37 Figure 1. PAH motifs. a) HB - anthracene [ANTCEN]. b) SHB – Quaterylene [QUATER10]. c) β-HB – 1, 2, 3, 4, 38 tetraphenylbenzene [FOVVOB]. d) γ – coronene [CORONE01]. e) β – anthra[2,1,9,8-hjkl ]benzo[de]naphtha 39 [2,1,8,7-stuv ]pentacene [BOXGAW]. 40 41 42 Despite the extensive knowledge of gas phase PAH band gap trends, little is known about 43 44 the band gap vs. structural/motif dependence in crystalline PAHs. We previously investigated 45 11, 12 46 the oligoacenes (a prototypical group of PAHs) in great detail using dispersion inclusive 47 density functional theory. In those studies (as well as others 13, 14 ) we benchmarked the 48 49 Tkatchenko-Scheffler dispersion energy method (PBE+vdW) for structural and properties 50 51 predictions of molecular crystals with a specific aim on the larger PAH family. The oligoacene 52 53 investigations mentioned above demonstrated that PBE+vdW can provide excellent structural 54 55 agreement with experiment, as well as accurately model the pressure induced structural changes 56 of naphthalene, anthracene, and pentacene. PBE+vdW was also used to reproduce the pressure 57 58 59 60 ACS Paragon Plus Environment The Journal of Physical Chemistry Page 4 of 36 1 2 3 induced phase transition of tetracene along with the pressure induced changes to the HOMO- 4 5 LUMO band gaps of tetracene and pentacene.
Details
-
File Typepdf
-
Upload Time-
-
Content LanguagesEnglish
-
Upload UserAnonymous/Not logged-in
-
File Pages37 Page
-
File Size-