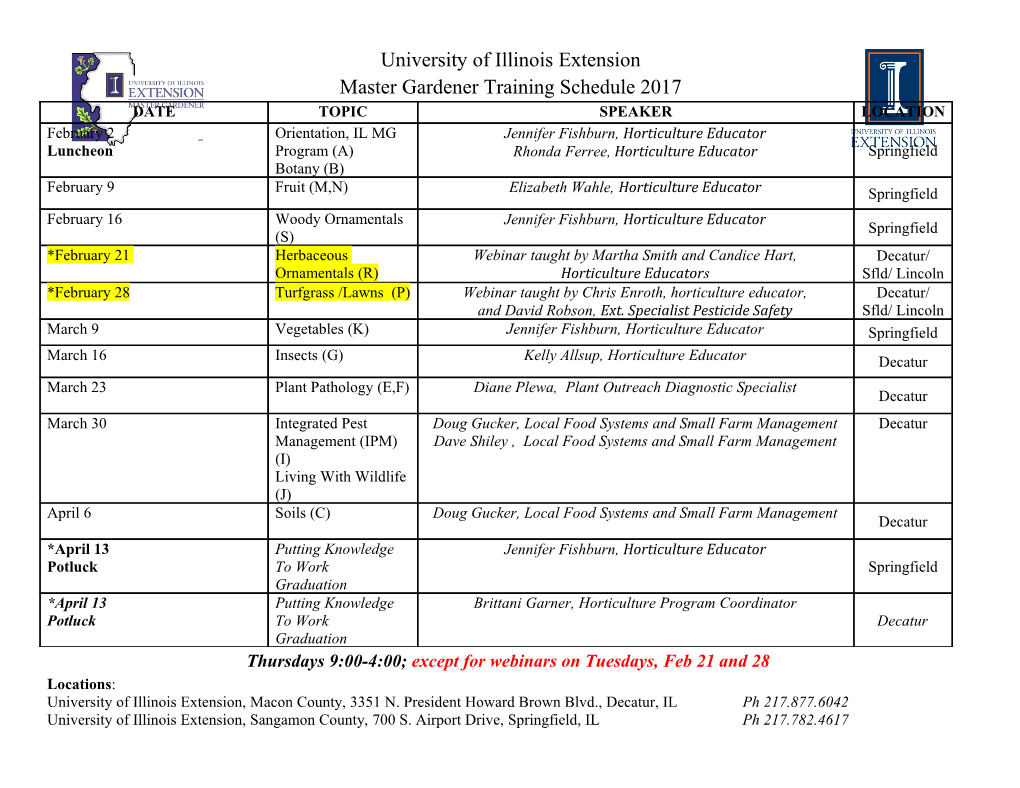
Atmospheric Environment xxx (2018) xxx-xxx Contents lists available at ScienceDirect Atmospheric Environment journal homepage: www.elsevier.com Detection of the dispersion and residence of volcanic SO2 and sulfate aerosol from Nabro in 2011 Yang Liua , b , Petri K.E. Pellikkac , d , e , Hansunbai Lia , b , Xiuqi Fanga , b , ∗ a Key Laboratory of Environmental Change and Natural Disaster of Ministry of Education, 100875, Beijing, China b Faculty of Geographical Science, Beijing Normal University, 100875, Beijing, China c College of Global Change and Earth System Science, Beijing Normal University, 1000875, Beijing, China d Earth Change Observation Laboratory, Department of Geosciences and Geography, University of Helsinki, P.O. Box 64, 00014, Helsinki, Finland e Institute for Atmospheric and Earth System Research, University of Helsinki, 00014, Helsinki, Finland PROOF ARTICLE INFO ABSTRACT Keywords: Continuous detection of dispersion and residence of volcanic plumes in troposphere and lower stratosphere is Volcanic SO2 vitally important for improving the understanding on the role of volcano eruptions in climate change. We report Diffusion path a 3-month continuous detection of dispersion and residence of volcanic plumes in the troposphere and stratos- Residence time phere generated from the volcanic SO2 erupted by Nabro in Eritrea on June 12th, 2011 observed by the OMI Evenly distribution sensor. The background SO concentration of 3 different height layers in troposphere and lower stratosphere Nabro 2 were estimated by the 3-year-average daily concentration of monthly SO2 in 2005, 2007 and 2013, when there were no large explosive volcanic eruptions occurring. We also traced the diffusion path and the concentration of volcanic SO2 for the first 3 months after Nabro's eruption, and detected the appearance and dissipation of sulfate aerosols, which is a product converted from volcanic SO2 . The results show that after Nabro erupted on June 12th, the volcanic plumes spread to middle latitudes (30°N-60°N) of Northern Hemisphere and loading by westerly jet. The volcanic SO2 in middle troposphere layer (TRM) and lower troposphere layer (TRL) stopped eastward spreading, and dissipated over the western Pacific Ocean on June 23rd. On June 26th, the volcanic SO2 in upper troposphere and lower stratosphere (STL) reached Mexico in Central America, and almost encircled the low latitudes and parts of middle latitudes. On June 28th, the volcanic SO2 plume showed an even distribution in STL. 37 days after the eruption, the volcanic SO2 in STL encircled the Northern Hemisphere evenly, sulfate aerosols in STL largely covered the low and middle latitudes and the daily concentration of SO2 was still higher than the background value. One month after the eruption, the global mean daily concentration of SO2 dropped to the normal value, but the daily concentration of SO2 and sulfate aerosols in low latitudes remained high, and dissipated not earlier than 3 months after the eruption of Nabro. ∗ Corresponding author. Key Laboratory of Environmental Change and Natural Disaster of Ministry of Education, 100875, Beijing, China. Email address: [email protected] (X. Fang) UNCORRECTED https://doi.org/10.1016/j.atmosenv.2018.10.022 Received 29 January 2018; Received in revised form 3 September 2018; Accepted 17 October 2018 Available online xxx 1352-2310/ © 2018. Y. Liu et al. Atmospheric Environment xxx (2018) xxx-xxx 1. Introduction tection of the dispersion area and residence period that volcanic SO2 could covering during the first date when Nabro erupted to the last date Volcanic eruption is one of the main natural processes injecting mas- that the plumes dissipated in different height layers are less focused on. sive ashes and gases into the troposphere and stratosphere (Robock, According to Global Volcanism Program (2013), SO2 emitted by 2000; Sakai et al., 2016). Apart from water vapor, sulfur dioxide (SO2 ) Nabro is one of the largest amount within the volcanic eruptions glob- is one of the main component of gases erupted by volcanoes (Wilson, ally during the last 20 years, following by the eruption of Pinatubo in 1985). Generally, several million tons of SO2 are injected into the at- 1991, which holds the largest emission event during the past century mosphere by an explosive volcanic eruption (Evans and Kerr, 1983; Guo (Theys et al., 2013; Robock et al., 2013; Fairlie et al., 2014). The sub- et al., 2004; Lettino et al., 2012); and remaining for several weeks or stantial SO2 yield from Nabro's eruption is estimated to be at around months in the upper troposphere and lower stratosphere (Robock, 2000; 4.5 Tg of which 1.3–2.0 Tg is into the upper troposphere and lower Fairlie et al., 2014; Mahmood et al., 2016). Due to long residence time stratosphere during the first 15 days of the eruption (Carn and Schwand- and large-scale dispersion in atmosphere, SO2 converts gradually into ner, 2012; Clarisse et al., 2014; Fromm et al., 2014). Santer et al. (2015) sulfate aerosol, which has a notable impact on local or global cooling and Goitom et al. (2011) argued that Nabro is one of the eruptions effect as well as climate change (Lamb, 1970; Hofmann et al., 2009). occurring during the last 20 years causing a significant effect on near Large explosive eruptions with Volcanic Explosivity Index (VEI) global troposphere temperature, sea surface temperature and precipita- higher than 4 are more likely to send its plume mass into the upper tion, and contributing to the changes on tropospheric temperature. troposphere and lower stratosphere (Hao et al., 2016). According to The aim of this study is to report a continuous detection of disper- the data published by Smithsonian Institute (Global Volcanism Program, sion area and residence time of volcanic SO2 and sulfate aerosols from 2013) about 75% of large explosive volcanic eruptions have reached up- Nabro in 2011. Using SO2 column data, sulfate data and wind data in per troposohere and lower stratosphere. Several volcanoes erupted after 3 different height layers in troposphere to lower stratosphere, the diffu- 2000 including Sarychev of Russia in 2006, Merapi of Indonesia in 2010 sion path and the process of evenly distribution of plumes after eruption and Nabro of Eritrea in 2011, had VEI grade 4. were traced. We aim to proPROOFvide the basic data for the studies about vol- Nabro's eruption took place on June 12th, 2011. Recent remote sens- canic impact. ing studies detected the components and aerosol optical depth (AOD) of eruption clouds, and identified the diffusion path of the clouds within 2. Eruption of Nabro in 2011 1–2 weeks after the eruption (Bourassa et al., 2012, 2013; Fromm et al., 2013; Vernier et al., 2013). Several studies carried out on Nabro's erup- Nabro's eruption on June 12th, 2011 is the first recorded eruption tion and its producsts. Clarisse et al. (2014) and Zerefos et al. (2017) of Nabro (Bojanowski, 2011). The volcano is located in a border area estimated the eruption height and the concentration of eruption com- of Eritrea and Ethiopia in the Horn of Africa (13.37°N, 41.7°E) with ponents. Hoffmann et al. (2016) and Heng et al. (2016) derived al- summit elevation of 2218 m above sea level (Fig. 1). The volcano is titude-resolved emission time series. Mahmood et al. (2016) and Noh part of a large massif called as the Bidu Volcanic Complex (Wiart and et al. (2017) analyzed the vertical distribution of volcanic plumes in Oppenheimer, 2005). The minimum plume altitude of Nabro on June the specific ground stations along the diffusion way. However, the de 13th was 18 km, with a total mass of 3650 kt based on the records from the Smithsonian Institute (http://volcano.si.edu). The eruption began before midnight on June 12th following sev- eral hours of ground shaking. The seismometers located in Ethiopia, Yemen and Djibouti did record Fig. 1. The plumes from Nabro over Ethiopia and Sudan, June 13th. UNCORRECTED 2 Y. Liu et al. Atmospheric Environment xxx (2018) xxx-xxx seismicity associated with the volcano's eruption, but they did not SO2 to sulfate aerosol over low and middle latitudes after Nabro's erup- provide information of operational value at the time of the eruption tion. The horizontal resolution of these data is 333 m; the vertical reso- (Goitom et al. 2011). Earthquakes occurring during several hours before lution in the height layer of 8–20 km above the surface is 60 m, and for the eruption prompted a rapid evacuation of settlements nearby, and the height lower than 8 km is 30 m (Vaughan et al., 2004). in total about 12,000 people were ultimately translocated (Solomon, 2012). Evacuation saved many lives, but seven people were found dead. 3.1.3. Wind field data The characteristics of the eruption and its consequences were analyzed Wind field data were obtained from National Center for Environ- and reported by Goitom et al. (2011) using optical satellite imagery ment Prediction (NCEP, http://www.esrl.noaa.gov). The data are grid such as ALI (Advanced Land Imager), SEVIRI (Spinning Enhanced Visi- data of daily mean zonal (u) and meridional (v) wind values with grid ble Infrared Imager), MODIS (Moderate Resolution Imaging Spectrora- unit of 2.5° × 2.5° and spatial range from 0.0 to 357.5E for longitude, diometer) and AVHRR (Advanced Very High Resolution Radiometer), and 90.0N to 90.0S for latitude (Kalnay et al., 1996). The wind database and SAR (Synthetic Aperture Radar) imagery such as TerraSAR-X and provides data on 17 standard pressure levels from 1000 mb to 10 mb. Cosmo-SkyMed. The plume from Nabro to the west over Ethiopia and Because the center heights of STL, TRM and TRL are 17 km, 7.5 km and Sudan is presented in a MODIS image captured on June 13th, 2011 in 3 km, respectively, wind field data on the height level of 100 mb (about Fig.
Details
-
File Typepdf
-
Upload Time-
-
Content LanguagesEnglish
-
Upload UserAnonymous/Not logged-in
-
File Pages10 Page
-
File Size-