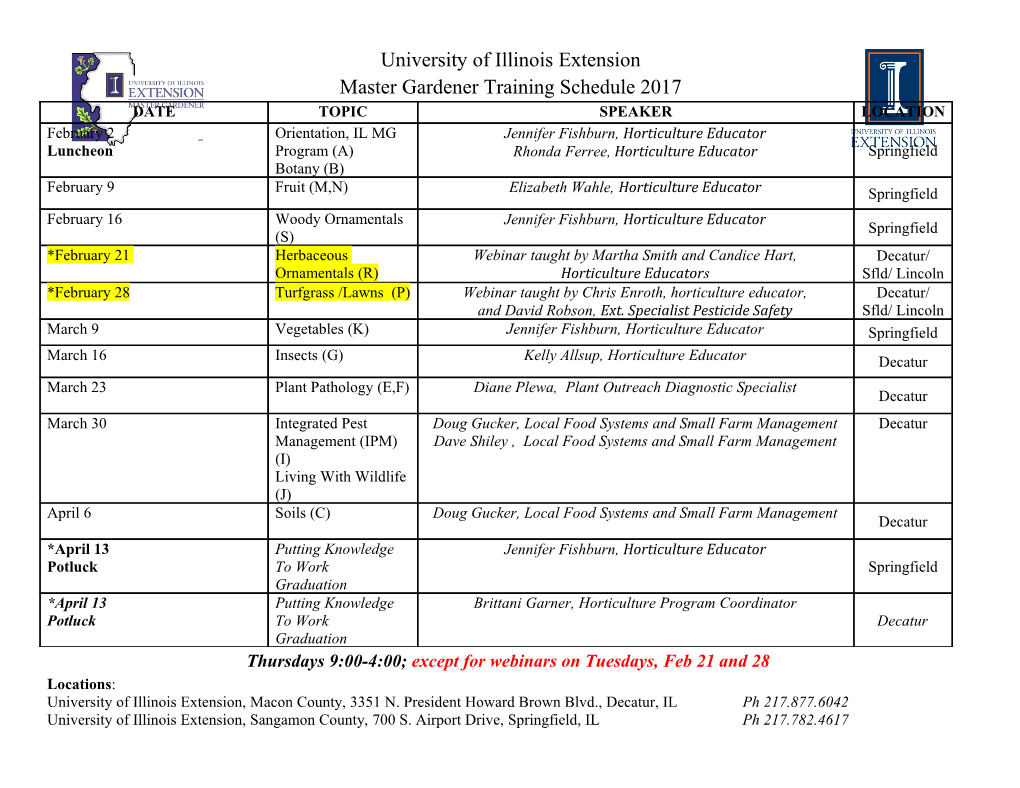
Energy & Environmental Science View Article Online REVIEW View Journal | View Issue Carbon capture and storage (CCS): the way forward Cite this: Energy Environ. Sci., 2018, 11,1062 ab bc d e Mai Bui, Claire S. Adjiman, Andre´ Bardow, Edward J. Anthony, Andy Boston,f Solomon Brown, g Paul S. Fennell,c Sabine Fuss,h Amparo Galindo,bc Leigh A. Hackett,i Jason P. Hallett, c Howard J. Herzog, j George Jackson,c Jasmin Kemper,k Samuel Krevor,lm Geoffrey C. Maitland, cl Michael Matuszewski,n Ian S. Metcalfe,o Camille Petit,c Graeme Puxty,p Jeffrey Reimer,q David M. Reiner,r Edward S. Rubin, s Stuart A. Scott,t Nilay Shah,bc Berend Smit, qu J. P. Martin Trusler,cl Paul Webley,vw Jennifer Wilcoxx and Niall Mac Dowell *ab Carbon capture and storage (CCS) is broadly recognised as having the potential to play a key role in meeting climate change targets, delivering low carbon heat and power, decarbonising industry and, more recently, its Creative Commons Attribution 3.0 Unported Licence. ability to facilitate the net removal of CO2 from the atmosphere. However, despite this broad consensus and its technical maturity, CCS has not yet been deployed on a scale commensurate with the ambitions articulated a decade ago. Thus, in this paper we review the current state-of-the-art of CO2 capture, transport, utilisation and storage from a multi-scale perspective, moving from the global to molecular scales. In light of the COP21 commitments to limit warming to less than 2 1C, we extend the remit of this study to include the key negative Received 17th August 2017, emissions technologies (NETs) of bioenergy with CCS (BECCS), and direct air capture (DAC). Cognisant of the Accepted 5th January 2018 non-technical barriers to deploying CCS, we reflect on recent experience from the UK’s CCS commercialisation DOI: 10.1039/c7ee02342a programme and consider the commercial and political barriers to the large-scale deployment of CCS. In all areas, we focus on identifying and clearly articulating the key research challenges that could usefully be This article is licensed under a rsc.li/ees addressed in the coming decade. Open Access Article. Published on 12 March 2018. Downloaded 10/11/2021 4:01:07 AM. a Centre for Environmental Policy, Imperial College London, South Kensington, London SW7 1NA, UK. E-mail: [email protected] b Centre for Process Systems Engineering, Imperial College London, South Kensington, London SW7 2AZ, UK c Department of Chemical Engineering, Imperial College London, South Kensington, London, SW7 2AZ, UK d Chair of Technical Thermodynamics, RWTH Aachen University, 52056 Aachen, Germany e Centre for Combustion, Carbon Capture & Storage, Cranfield University, Bedford, Bedfordshire MK43 0AL, UK f Red Vector Ltd., Barrow Upon Soar, Loughborough, Leics LE12 8JY, UK g Department of Chemical and Biological Engineering, The University of Sheffield, Sheffield S1 3JD, UK h Mercator Research Institute on Global Commons and Climate Change, 10829 Berlin, Germany i Industria Mundum AG, Zu¨rich, Switzerland j Massachusetts Institute of Technology, Cambridge, Massachusetts 02139, USA k IEA Greenhouse Gas R&D Programme (IEAGHG), Pure Offices, Cheltenham Office Park, Hatherley Lane, Cheltenham, Gloucestershire, GL51 6SH, UK l Qatar Carbonates and Carbon Storage Research Centre, Department of Chemical Engineering, Imperial College London, South Kensington Campus, London SW7 2AZ, UK m Department of Earth Science & Engineering, Imperial College London, South Kensington, London, SW7 2AZ, UK n US Department of Energy, National Energy Technology Laboratory (NETL), Pittsburgh, PA, USA o School of Chemical Engineering and Advanced Materials, Newcastle University, Newcastle-upon-Tyne NE1 7RU, UK p CSIRO Energy, Mayfield West, New South Wales 2304, Australia q Department of Chemical and Biomolecular Engineering, University of California Berkeley, Berkeley, CA 94720, USA r Energy Policy Research Group, Judge Business School, University of Cambridge, Cambridge, UK s Engineering & Public Policy and Mechanical Engineering, Carnegie Mellon University, Pittsburgh, Pennsylvania 15213, USA t University of Cambridge, Department of Engineering, Cambridge CB2 1PZ, UK u Laboratory of Molecular Simulation, Institut des Sciences et Inge´nierie Chimiques, Valais Ecole Polytechnique Fe´de´rale de Lausanne (EPFL), Rue de l’Industrie 17, CH- 1951 Sion, Switzerland v Department of Chemical Engineering, The University of Melbourne, Victoria 3010, Australia w The Peter Cook Centre for Carbon Capture and Storage, The University of Melbourne, Victoria 3010, Australia x Chemical and Biological Engineering Department, Colorado School of Mines, Golden, Colorado 80401, USA 1062 | Energy Environ. Sci., 2018, 11, 1062--1176 This journal is © The Royal Society of Chemistry 2018 View Article Online Review Energy & Environmental Science Broader context Carbon capture and storage (CCS) is recognised as being vital to least cost pathways for climate change mitigation, and in particular the negative emissions technologies (NETs) that are key to limiting warming to ‘‘well below’’ 2C. However, it has not yet been deployed on the scale understood to be required, owing to a variety of technical, economic and commercial challenges. This paper provides a state-of-the-art update of each of these areas, and provides a perspective on how to the discipline forward, highlighting key research challenges that should be addressed over the course of the next decade. Importantly, this perspective balances scientific, policy and commercial priorities. 1 Introduction capital and operating cost of the processes in which they are used. For this reason, there is a concerted effort to rationally This paper is the third installment in a series of publications over design new sorbent materials, with the bulk of the effort in the several years in Energy & Environmental Science.1,2 The first development of liquid sorbents, where available theories are (published in 2010) provided an introduction to CO2 capture more readily applied. Thus, we present an assessment of SAFT- technologies, with an overview of solvent-based chemisorption based approaches to model and design new materials in (amines and ionic liquids), carbonate looping, oxy-fuel combus- Section 6, with a focus on how efforts at the molecular and tion technologies, CO2 conversion and utilisation (CCU) and multi- process scales might be linked. 1 scaleprocessengineeringofCCS. The second installment pre- Before CO2 can be safely and reliably sequestered, it must be sented an update on developments in amine scrubbing, ionic transported from source to sink. Whilst the majority of studies liquids, oxy-combustion and calcium looping. New topics added in assume pipeline transport, ship and rail transport are potential this second paper include chemical looping combustion, low alternatives; these other transport options are discussed in temperature adsorbents, direct air capture technologies, flexible Section 7. Similarly, despite the fact that CO2 transport by Creative Commons Attribution 3.0 Unported Licence. CCS operation, CO2 transport and storage, and a historical over- pipeline is exceptionally mature, the impact of capturing CO2 view of the UK and EU CCS policy and legislation.2 from a diverse set of power and industrial sources on the Distinct from the previous installments, this third paper sets out quality of CO2 being transported is sufficiently important to to comprehensively review the state-of-the-art developments in CCS, warrant careful consideration. whilst also providing a holistic perspective on the role of CCS The typical fate of CO2 is to be sequestered, either in a saline technologies in mitigating anthropogenic climate change. We first aquifer or, potentially, used for enhanced oil recovery (EOR). The discuss the current status of CCS development and highlight key various challenges of operation, monitoring and verification of CO2 CCS technologies that are near commercialisation phase (Section 2). storage are discussed in Section 8, whereas Section 9 discusses Then in Section 3 we contextualise CCS technology by considering its CO2-EOR. A potential alternative to the storage of CO2 is its re-use – This article is licensed under a representation and utilisation in integrated assessment models the valorisation of CO2 to produce marketable compounds. The (IAMs), challenging the view that it is a ‘‘bridging technology’’, likely argument is sometimes made that this can both contribute to to be relevant for only a few decades. We then go on to quantify and climate change mitigation and provide an attractive revenue stream. Open Access Article. Published on 12 March 2018. Downloaded 10/11/2021 4:01:07 AM. qualify the role and value of CCS at a more granular level by Section 10 discusses the potential for CO2 conversion and utilisation evaluating the way in which CCS interacts with national scale (CCU), also its merits and challenges are presented and considered. electricity systems. This in turn helps us address the question of In light of the global commitment achieved in Paris in what service CCS provides to the electricity system, with whom is December, 2015,3 we have extended this paper to include key CCS competing and what technologies does CCS complement. negative emissions technologies (Section 12); bioenergy with We then move on to consider the utility of CCS in decarbonis- CCS (BECCS) and direct air capture of CO2 (DAC). These areas ing the industrial sector, with a focus on the key emitters – the are of particular importance owing to their potential impor-
Details
-
File Typepdf
-
Upload Time-
-
Content LanguagesEnglish
-
Upload UserAnonymous/Not logged-in
-
File Pages115 Page
-
File Size-