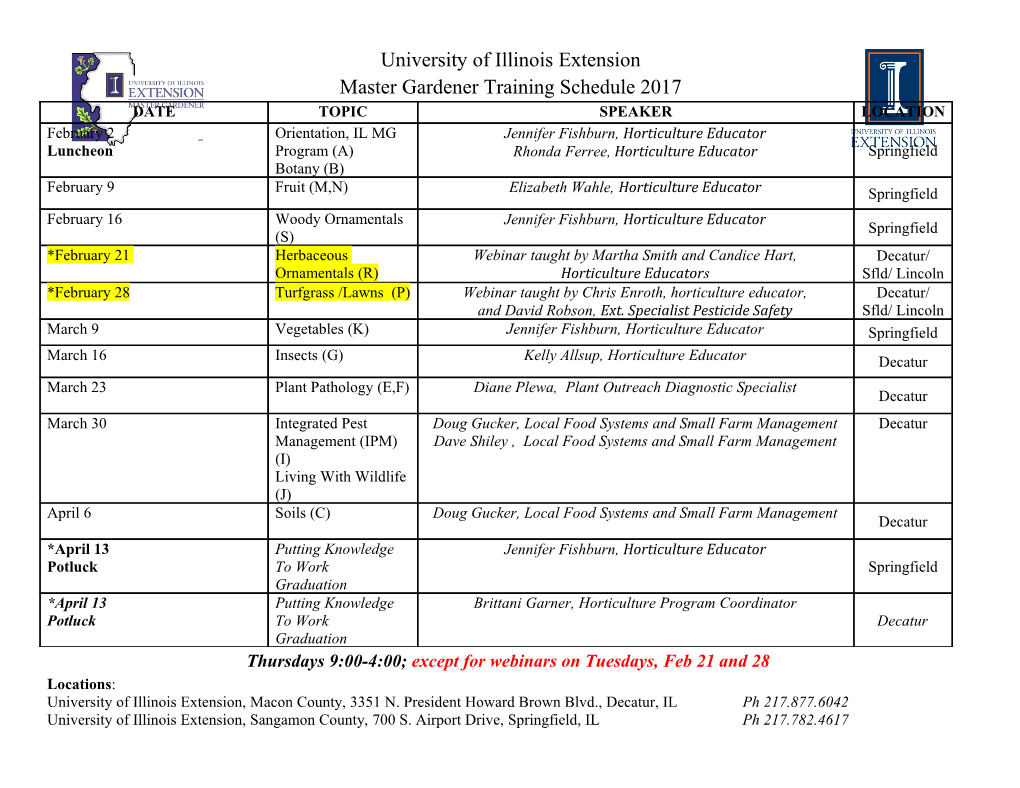
This may be the author’s version of a work that was submitted/accepted for publication in the following source: Cox, Kelly, Renouf, Marguerite, Dargan, Aidan, Turner, Christopher, & Klein-Marcuschamer, Daniel (2014) Environmental life cycle assessment (LCA) of aviation biofuel from microal- gae, Pongamia pinnata, and sugarcane molasses. Biofuels, Bioproducts and Biorefining, 8(4), pp. 579-593. This file was downloaded from: https://eprints.qut.edu.au/205157/ c 2014 Society of Chemical Industry and John Wiley and Sons, Ltd This work is covered by copyright. Unless the document is being made available under a Creative Commons Licence, you must assume that re-use is limited to personal use and that permission from the copyright owner must be obtained for all other uses. If the docu- ment is available under a Creative Commons License (or other specified license) then refer to the Licence for details of permitted re-use. It is a condition of access that users recog- nise and abide by the legal requirements associated with these rights. If you believe that this work infringes copyright please provide details by email to [email protected] Notice: Please note that this document may not be the Version of Record (i.e. published version) of the work. Author manuscript versions (as Sub- mitted for peer review or as Accepted for publication after peer review) can be identified by an absence of publisher branding and/or typeset appear- ance. If there is any doubt, please refer to the published source. https://doi.org/10.1002/bbb.1488 1 Environmental life cycle assessment (LCA) of aviation biofuel from microalgae, 2 Pongamia pinnata, and sugarcane molasses 3 Kelly Cox, Boeing Research and Technology Australia, Brisbane Technology Centre, Brisbane, 4 QLD, Australia. 5 Marguerite Renouf, University of Queensland, School of Geography, Planning and Environmental 6 Management, St. Lucia, QLD, Australia. 7 Aidan Dargan, Boeing Research and Technology Australia, Brisbane Technology Centre, Brisbane, 8 QLD, Australia. 9 Christopher Turner, University of Queensland, Australian Institute of Bioengineering and 10 Nanotechnology, St. Lucia, QLD, Australia. 11 Daniel Klein-Marcuschamer, University of Queensland, Australian Institute for Bioengineering 12 and Nanotechnology, St Lucia, Australia; Joint BioEnergy Institute, Deconstruction Division, 13 Emeryville, CA, USA; Lawrence Berkeley National Laboratory, Physical Biosciences Division, 14 Berkeley, CA, USA 15 Correspondence to: Kelly Cox, Boeing Research and Technology Australia, Brisbane, QLD, 16 Australia. 17 E-mail: [email protected] 18 19 ABSTRACT 20 The environmental benefits and trade-offs of automotive biofuels are well known, but less is known 21 about aviation biofuels. We modeled the environmental impacts of three pathways for aviation 22 biofuel in Australia (from microalgae, pongamia, and sugarcane molasses) using attributional life 23 cycle assessments (LCA), applying both economic allocation and system expansion. Based on 24 economic allocation, sugarcane molasses has the better fossil energy ratio FER (1.7 MJ out/MJ in) 25 and GHG abatement (73% less than aviation kerosene) of the three, but with the trade-offs of higher 26 water use and eutrophication potential. Microalgae and pongamia have lower FER and GHG 1 1 abatement (1.0 and 1.1; 53% and 43%), but mostly avoid the eutrophication and reduce the water use 2 trade-offs. All have similar and relatively low land use intensities. If produced on land where existing 3 carbon stocks are not compromised, the sugarcane and microalgae pathways would currently meet a 4 50% GHG abatement requirement, and some GHG mitigation would be required for the pongamia 5 pathway to achieve this. This is reasonably promising given the early developmental status of these 6 pathways. Based on system expansion, microalgae and pongamia pathways had lower impacts than 7 the sugarcane pathway for all categories except energy input, highlighting the positive aspects of 8 these “next–generation” feedstocks. The low fossil energy conservation potentials of these pathways 9 was found to be a, and significant energy efficiencies will be needed before they can effect fossil 10 energy conservation. Energy recovery from processing residues (base case) was preferable over their 11 use as animal feed (variant case), and crucial for favourable energy and GHG conservation. However 12 this finding is at odds with the economic preferences identified in a companion technoeconomic 13 study. 14 15 Supporting information can be found in the online version of this article. 16 Keywords: LCA; environmental impact; energy; greenhouse gas; alternative fuel; jet fuel; 17 18 1. INTRODUCTION 19 The aviation industry contributes around three percent of the radiative forcing from energy-related 20 greenhouse gas (GHG) emissions.1 The International Air Transport Association (IATA) has set a 21 target to halve emissions from the global aviation sector by 2050 compared with 2005 levels, and one 22 strategy is the use of aviation biofuels (IATA (www.iata.org)). While biofuels alone are not expected 23 to achieve this target, they can make a substantial contribution depending on the feedstock and 24 production pathway.2 Identification and development of optimal GHG saving pathways will require 25 collaboration between government, the aviation industry, research organisations and fuel 26 manufacturers. The Queensland Sustainable Aviation Fuel Initiative (QSAFI) project is such a 27 collaboration established to assess the feasibility of three pathways for aviation biofuels in Australia, 2 1 based on molasses from sugarcane (Saccharum spp.), photoautotrophic microalgae (Nannochloropsis 2 spp.) and pongamia seeds (Pongamia pinnata). The focus was on aviation turbine fuels. A 3 companion technoeconomic study has been published previously in this journal.3 The current work 4 assesses the environmental impacts of the pathways using environmental life cycle assessment 5 (LCA) based on the same process models, data and assumptions as the technoeconomic study 6 (QSAFI (www.aibn.uq.edu.au/qsafi)). 7 Past LCA studies of biofuels generally have found them to offer fossil energy conservation and GHG 8 mitigation, but the extent varies depending on the feedstock and the production pathway, and the 9 assessment methodology applied.4-7 Greater recognition of GHG emissions associated with land use 10 change has introduced caution for first-generation biofuels that necessitate expanded agricultural 11 production.5, 8 Impacts from nitrogen use have also been highlighted, both in regards to emissions of 9-12 12 nitrous oxide (N2O) and eutrophication potential. Other issues are increased stress on water 13 resources13-15 and land use change (both direct and indirect) in relation to the loss of eco-system 14 services16 and competition with food production.17, 18 With increasing recognition of the 15 environmental constraints of large-scale bio-production,19, 20 and the development of environmental 16 criteria for future investment in bio-production,21, 22 it is important for environmental implications of 17 new biofuel pathways to be fully understood so that their GHG abatement potential can be harnessed 18 sustainably. 19 The aim of this study was to evaluate the life cycle environmental impacts of the three selected 20 production pathways, to test if they are sustainable alternatives to their fossil-fuel equivalent. This 21 was done by comparing the (expected) GHG savings relative to aviation kerosene with current GHG 22 abatement standards,21, 22 and by considering other environmental impacts (land use, water use, 23 eutrophication, ecotoxicity). The environmental assessment was also considered alongside the 24 companion technoeconomic assessment3 to draw out synergies and conflicts between economic and 25 environmental objectives. 26 The three assessed feedstocks are promising because they can be produced in commercial quantities 27 under Queensland conditions. Sugarcane molasses is a by-product of producing raw sugar from 3 1 sugarcane, a major commercial crop in Queensland. Molasses is preferred over cane juice as a 2 fermentation substrate in the Australian context as it reduces diversion of sucrose away from raw 3 sugar production, which is the industry’s core focus. Pongamia is a leguminous, oilseed tree native 4 to India that has become naturalized in Australia. While not yet domesticated or grown 5 commercially, it has been the subject of research because of its potential to produce commercial 6 quantities of oil in Australian conditions.23 Microalgae are a family of simple-structured 7 photosynthetic organisms with high photosynthetic efficiency and lipid content, which can be 8 converted into microalgae oil. Microalgae are promising because they have the potential for high 9 biomass productivity per unit area, they can be grown without the need for arable land or fresh water, 10 and can utilise seawater, brackish waters and wastewaters.24 The engineering processes for producing 11 aviation fuel modelled in this study are based on a suite of technologies currently being investigated 12 in Queensland, and described further in 2.2. They do not represent aviation biofuel production in 13 general, and are in the early stages of development. 14 The production of first- and second-generation biofuels from sugarcane has been extensively 15 assessed using LCA. Sugarcane has consistently been shown to be amongst the best energy and 16 GHG saver relative to other feedstocks,4, 6, 7 but the first-generation
Details
-
File Typepdf
-
Upload Time-
-
Content LanguagesEnglish
-
Upload UserAnonymous/Not logged-in
-
File Pages25 Page
-
File Size-