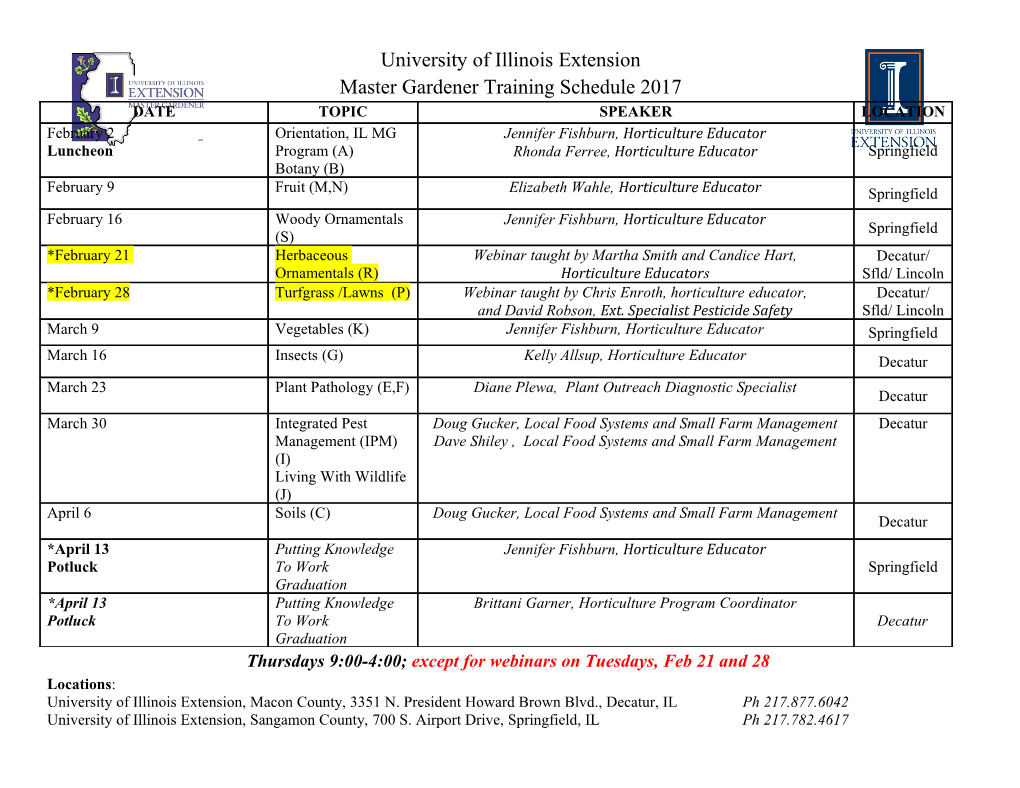
Nuclear Instruments and Methods in Physics Research A 705 (2013) 36–41 Contents lists available at SciVerse ScienceDirect Nuclear Instruments and Methods in Physics Research A journal homepage: www.elsevier.com/locate/nima Measurement of internal conversion electrons from Gd neutron capture P. Kandlakunta, L.R. Cao n, P. Mulligan Nuclear Engineering Program, Department of Mechanical and Aerospace Engineering, The Ohio State University, Columbus, OH 43210, USA article info abstract Article history: Gadolinium (Gd) is a suitable material for neutron conversion because of its superior neutron Received 21 September 2012 absorption cross-section. However, the principal secondary particles that generate electron-hole pairs Accepted 10 December 2012 in a semiconductor detector after Gd neutron capture are low-energy internal conversion (IC) electrons. Available online 20 December 2012 We measured the IC electron spectrum due to Gd neutron capture by using a thermal neutron beam Keywords: and a digitizer-based multidetector spectroscopy. We also discussed the effective use of the IC electrons Gadolinium neutron absorption in the context of a twin-detector design and the associated gamma-ray rejection issues. Extensive Gamma-ray rejection simulations of the spectra of IC electrons and gamma rays agreed well with the experimental results; Internal conversion electrons both types of results support the feasibility of the proposed n–g separation method. Semiconductor & 2012 Elsevier B.V. All rights reserved. 1. Introduction release of an average of 3.288 photons. These photons have a wide range of energies with a mean of 2.394 MeV [5]. A large number Compressed 3He gas is widely used as the standard medium of internal conversion (IC) electrons are emitted owing to the for detecting neutrons, primarily because of its large thermal large change in the angular momentum of the low-lying excited neutron cross-section and insensitivity to gamma radiation. The states of 158Gd*. Following IC, the Gd atom relaxes to the ground high demand for 3He in homeland security and nonproliferation state by emitting Auger electrons and characteristic X-rays. In this applications, and its widespread use in areas such as cryogenics study, we focus on the use of Si detectors to capture IC electrons [1], spallation neutron sources [2], and magnetic resonance created by neutron capture in Gd. imaging [3] have caused a long-standing shortage of 3He. Thus, Although abundant prompt gamma rays are emitted from an a replacement is urgently needed. Alternatives in which 10Bor6Li excited Gd* nucleus [6], they are mostly of high energy and compounds are applied as coatings are fundamentally unable to therefore almost transparent to a thin-film semiconductor detec- achieve a high intrinsic efficiency because of the conflict between tor. Thus, IC electrons are the principal neutron signal generator the comparatively long neutron mean free paths relative to the in the detector. In this study, we measured the IC electron energy short ranges of charged particles in the neutron conversion spectrum using a newly built 10 mm diameter thermal neutron media. As a low-density gas, 3He is less constrained by this beam at the Ohio State University Research Reactor (OSURR) conflict. This problem could be overcome by using gadolinium and digitizer-based multidetector spectroscopy system [7].We (Gd) as a neutron convertor because of its extremely high cross- also discuss the effective use of the IC electrons in the context of a section and Q value [4].The two most abundant isotopes of Gd and twin-detector design and the associated gamma-ray rejection their corresponding nuclear reactions induced by thermal neu- issues. trons are described in the following equations: 157 -158 n-158 nþ 64 Gd 64 Gd 64 Gdþgþconversion electronþ7:9 MeV, 2. IC electrons and range s0 ¼ 253,929 b ð1Þ n The most intense IC electrons emitted during de-excitation of nþ 155Gd-156Gd -156Gdþgþconversion electronþ8:5 MeV, 64 64 64 158Gd* and 156Gd* are listed in Table 1. The total yield of IC s0 ¼ 60,800 b ð2Þ electrons per neutron capture in Gd is 0.5991, as suggested by Harms and McCormack [8].The IC electrons do not have a wide 157 Following the absorption of a neutron by the Gd nucleus, for spectrum of energies and come primarily from the lowest two example, several isomeric transitions occur, which result in the energy-level transitions of the excited 158Gd* nucleus. Depending on whether the IC is on the K, L, or M shell, electrons are emitted n Corresponding author. Tel.: þ1 614 247 8701. in the energy range of 29–246 keV, with the most intense electron E-mail address: [email protected] (L.R. Cao). emission occurring at 71 keV. 0168-9002/$ - see front matter & 2012 Elsevier B.V. All rights reserved. http://dx.doi.org/10.1016/j.nima.2012.12.077 P. Kandlakunta et al. / Nuclear Instruments and Methods in Physics Research A 705 (2013) 36–41 37 Table 1 literature [10–13]. The continuous slowing down approximation IC electrons emitted during the de-excitation of Gd* nucleus [8]. (CSDA) estimates the electron range as the average of the total path length traveled by an electron as it moves through the IC electron energy (keV) Yield per IC electron energy (keV) Yield per from 158Gd* neutron from 156Gd* neutron material. The maximum penetration depth (MPD) of an electron is the straight-line distance between the beginning of the electron’s 29 0.0982 39 0.0419 track and its end. We evaluated the ranges of IC electrons from 71 0.268 81 0.0497 158Gd*, in various definitions, in Gd, Si, and polyethylene, both 78 0.0617 88 0.0116 theoretically and by Monte Carlo simulations. The CSDA was 131 0.0341 149 0.0084 173 0.0146 191 0.003 computed by the integral of the inverse stopping power [11] and 180 0.0031 198 0.0006 two Monte Carlo codes were used, i.e., the Penetration and Energy 228 0.004 246 0.0002 Loss of Positrons and Electrons (PENELOPE) [14] for the average Total yield 0.4837 Total yield 0.1154 path length and the Monte Carlo Simulation of Electrons Trajec- tory in Solids (CASINO) [15] for the MPD. All the values are tabulated in Table 2. The theoretical CSDA values of the electron range in these materials were computed using an effective atomic number and effective atomic weight for the material. The analytical expres- sions for both were extracted from Tabata et al [12]. As shown in Table 2, the average path lengths of electrons in the material are always larger than the MPD owing to multiple scattering deflec- tions [16]. The theoretical values and the average path length derived from PENELOPE agree because both deal with the total path length. As an example, the distribution of the MPD and the average path length of 71 keV electrons (from 158Gd*)ina50mm-thick Si detector are shown in Fig. 2. Si was chosen because the range of IC electrons in the detector material is the most important Fig. 1. Gamma-ray rejection scheme with a twin-detector configuration, a Gd foil, parameter. In contrast to a detector in which 10Bor6Li is applied, and a polyethylene layer. where the neutron-induced charged particles follow a straight, short path, the effective path length of electrons in a detector (e.g. Although the small volume and low Z of a thin-film semi- Si) is twice as long as its average MPD. This indicates a longer conductor detector make it relatively insensitive to external gamma rays, those low-energy external gamma rays that fall Table 2 within the IC electron energy range could lead to false positive Ranges of IC electrons in Si, Gd, and polyethylene emitted in Gd neutron capture neutron detection. One such application where this could present according to various range definitions (all units in mm). an issue is in special nuclear material detection, which typically occurs in environments that are ‘‘neutron signal starved’’, but Energy Gadolinium Silicon Polyethylene ‘‘gamma signal abundant’’ [9]. To mitigate the likelihood of a false (keV) Av. CSDA Av. Av. CSDA Av. Av. CSDA Av. detection due to gamma rays, a twin-detector scheme is proposed path MPD path MPD path MPD using Gd as a neutron convertor, and two detectors to identify length length length and reject external gamma rays, as shown in Fig. 1. Rejection is achieved by introducing a layer of Gd foil and another layer of an 29 4.47 4.95 1.2 9.33 9.80 4.13 16.3 18.6 10.7 71 20.1 23.4 5.03 43.8 49.2 21.0 79.1 95.3 54.8 electron separator material (polyethylene) into the composite 78 23.5 27.6 5.90 51.4 58.4 24.7 93.1 113 64.8 detector scheme. Detector 1 is placed in direct contact with a Gd foil, allowing the detection of IC and Auger electrons, as well as gamma rays produced by neutron absorption in Gd. A second detector is placed on the back side of the Gd layer, but uses a polyethylene layer of appropriate thickness to stop all Gd gener- ated IC and Auger electrons from reaching detector 2. In this configuration, detector 2 is sensitive only to gamma rays. Hence, detector 1 generates a combined signal induced by both neutrons and gamma rays, whereas detector 2 produces a signal induced only by gamma rays. Subtracting the two detector signals would yield a net signal induced solely by neutrons. Silicon (Si) detectors were used in the following experiments and simulations, but other semiconductor materials could be used, e.g., gallium nitride.
Details
-
File Typepdf
-
Upload Time-
-
Content LanguagesEnglish
-
Upload UserAnonymous/Not logged-in
-
File Pages6 Page
-
File Size-