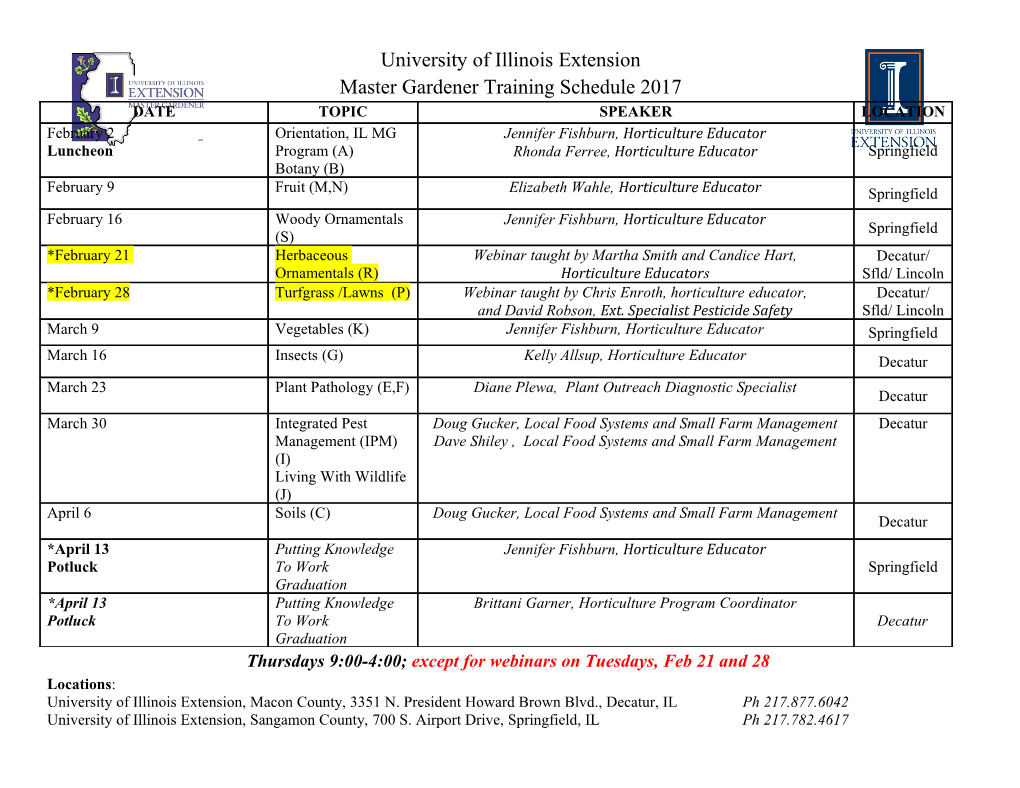
INTEGRATION OF PROTEIN PROCESSING STEPS ON A DIGITAL MICROFLUIDICS PLATFORM FOR ANALYSIS BY MALDI-MS Debalina Chatterjee,1 Anders J. Ytterberg,2 Sang Uk Son,2 Joseph A. Loo2,3 and Robin L. Garrell1,2,3 1Biomedical Engineering Program, University of California Los Angeles, CA USA 2Department of Chemistry and Biochemistry & 3California NanoSystems Institute, University of California Los Angeles, CA USA ABSTRACT A droplet-based (digital) microfluidic platform has been used to process protein samples for matrix-assisted laser desorption/ionization mass spectrometry (MALDI- MS) analysis. The steps include dispensing all reagents and performing protein reduction, alkylation and enzymatic digestion, crystallization of the resulting peptides with MALDI matrix, and in situ MALDI-MS analysis. The method is fast, simple, repeatable, and results in lower reagent consumption and sample loss than conventional techniques for proteomics sample preparation. KEYWORDS: Droplet, Microfluidics, Electrowetting, MALDI-MS EXPERIMENTAL The droplet microfluidics platform used to process proteomics samples for MALDI-MS analysis is similar to one described previously (Fig. 1) [1,2]. Droplets of liquid are moved in air by sequentially applying an electric potential to an array of electrodes patterned beneath a hydrophobic dielectric layer [3]. The electromechanical force actuates droplets by electrowetting-on-dielectrics (EWOD), dielectrophoresis, or a combination of the two mechanisms. It was previously shown that multiple droplets could be dispensed sequentially for MALDI-MS analysis [4]. On-chip reduction and digestion have also been shown [5]. Here, we demonstrate integration of all protein processing steps for MALDI-MS. Key modifications over previously ITO ground electrode glass substrate used devices are the incorporation of Teflon four on-chip reservoirs and the selective use of Teflon-AF and parylene glass substrate coatings. Droplet evaporation on Teflon dielectric layer (parylene) Cr/Au driving electrode yields dense crystals but few good droplet movement area reaction area spectra. Because parylene is less Figure 1: Schematic device cross-section. hydrophobic, droplets spread out and evaporate faster, generating small homogenous crystals from which spectra are easily obtained. Fig. 1 shows the device partially coated with Teflon to facilitate droplet movement. The parylene surface serves as the reaction/detection site. Droplets containing the analyte, bovine serum albumin (BSA) or lysozyme, and the reducing agent TCEP (tris-2- carboxyethyl phosphine hydrochloride) were merged and allowed to react at R.T. for 5 min. A third droplet containing the alkylating reagent, NEM (N-ethylmaleimide) Twelfth International Conference on Miniaturized Systems for Chemistry and Life Sciences October 12 - 16, 2008, San Diego, California, USA 978-0-9798064-1-4/µTAS2008/$20©2008CBMS 1072 was transported to the reduced analyte and allowed to react for 5 min. A fourth droplet containing the proteolytic enzyme trypsin was transported to the reduced, alkylated analyte and allowed to react for 4 h in an incubator (37 ˚C). Finally, a droplet containing matrix, α-cyano-4-hydroxycinnamic acid (CHCA), was dispensed into the processed analyte droplet and allowed to dry in air. The device (30×15×0.5 mm) was affixed to a custom MALDI target and inserted into a Voyager DE STR spectrometer (Applied Biosystems). MALDI spectra were collected in reflector mode. Peaks were searched against the SwissProt database by the Mascot protein identification search engine [6] using the following parameters: trypsin with max 1 miscleavage, variable methionine oxidation and cysteine alkylation, and an error tolerance of 100 ppm. RESULTS While proteins lacking disulfide bonds are easily digested using trypsin [5], proteins with disulfide bonds need to be reduced prior to digestion. Reduction allows the protein to unfold, maximizing enzyme access to cleavage sites. An alkylating agent is used to prevent the thiol groups from reforming disulfide bonds. Lysozyme and BSA were chosen to demonstrate the combined on-chip sequence of reduction, alkylation, and digestion. Figure 2 shows the peptide mass fingerprint (PMF) for chicken egg lysozyme. Using internal calibration, the search yielded sequence coverage of 74% and a relatively high Mowse score Full spectrum of 214. The limit for Lysozyme: 2.5 significant identification at pmol on-target P<0.05 was 68, with 21 matching peptides and less than a 40 ppm error. All major peaks could be assigned to lysozyme. For the BSA PMF analysis, the sequence coverage was 37% and Mowse score 159. The limit for significant identifica- tion at P<0.05 was 68, with Figure 2. Lysozyme PMF showing 23 matching 25 matching peptides and <40 peptide ions. Peptides with cysteine residues are ppm error. All the major indicated by “+” if alkylated or “-” if not. peaks except one could be matched to BSA. Most of the protein molecules were fully cleaved or had one miscleavage. In general, where miscleavage occurred, there were either two adjacent cleavage sites or the cleavage site was adjacent to an acidic residue, which is known to cause miscleavages under the best reaction conditions. There were very few unidentified peaks (none for lysosyme, two minor for BSA) suggesting no or only minor side products. Data collected from different trials involving the same protein yielded some variation in signal intensity but the overall pattern was similar, as illustrated in Figure 3. Peaks of the same M/Z were found in each spectrum and the peaks had Twelfth International Conference on Miniaturized Systems for Chemistry and Life Sciences October 12 - 16, 2008, San Diego, California, USA 1073 approximately the same relative intensities. Preliminary results for protein mixtures show that reduction, alkylation and digestion are not affected by greater sample complexity. * 1 - * 2 3 4 980 m/z 1140 Figure 3. Partial MALDI-MS spectra (m/z range 980 to 1140) from replicate on-chip lysozyme digestions. - indicates free cysteine and * indicates alkylated cysteine. CONCLUSIONS Droplet microfluidics can be used for fully integrated protein sample preparation for MALDI-MS. Potential applications include method optimization and general proteomics sample preparation. ACKNOWLEDGMENTS Christopher Bird and Ivory Peng contributed to early phases of this work. Support from CMISE, a NASA URETI at UCLA (award #NCC 2-1364), the NSF IGERT Materials Creation Training Program (DGE0654431), and the National Institutes of Health (RR020070) is gratefully acknowledged. REFERENCES [1] "Electrowetting-Based Microfluidics for Analysis of Peptides and Proteins by Matrix Assisted Laser Desorption/Ionization Mass Spectrometry (MALDI-MS) ", A. R. Wheeler, H. Moon, C.-J. Kim, J. A. Loo, R. L. Garrell, Analytical Chemistry, 76, 4833 (2004). [2] "Digital microfluidics with in-line sample purification for proteomics analyses with MALDI-MS", A. R. Wheeler, H. Moon, C. A. Bird, R. R. O. Loo, C.-J. Kim, J. A. Loo, R. L. Garrell, Analytical Chemistry, 77, 534 (2005). [3] "Droplet based Microfluidics with Nonaqueous Solvents and Solutions", D. Chatterjee, B. Hetayothin, A. R. Wheeler, D. J. King, R. L. Garrell, Lab on a Chip, 6, 199 (2006). [4] "Integrated digital microfluidic chip for multiplexed proteomic sample preparation and analysis by MALDI-MS", H. Moon, A. R. Wheeler, R. L. Garrell, J. A. Loo, C.-J. Kim, Lab Chip, 6, 1213 (2006). [5] "Digital Microfluidics Based Method for Proteomics", A. R. Wheeler, C. A. Bird, D. Chatterjee, H. Moon, R. R. O. Loo, C.-J. Kim, J. A. Loo, R. L. Garrell, MicroTAS, USA, 2, 782 (2005). [6] "Probability-Based Protein Identification by Searching Sequence Databases Using Mass Spectrometry Data", D.N. Perkins, D.J.C. Pappin, D.M. Creasy and J.S. Cottrell, Electrophoresis, 20, 3551(1999). Twelfth International Conference on Miniaturized Systems for Chemistry and Life Sciences October 12 - 16, 2008, San Diego, California, USA 1074.
Details
-
File Typepdf
-
Upload Time-
-
Content LanguagesEnglish
-
Upload UserAnonymous/Not logged-in
-
File Pages3 Page
-
File Size-