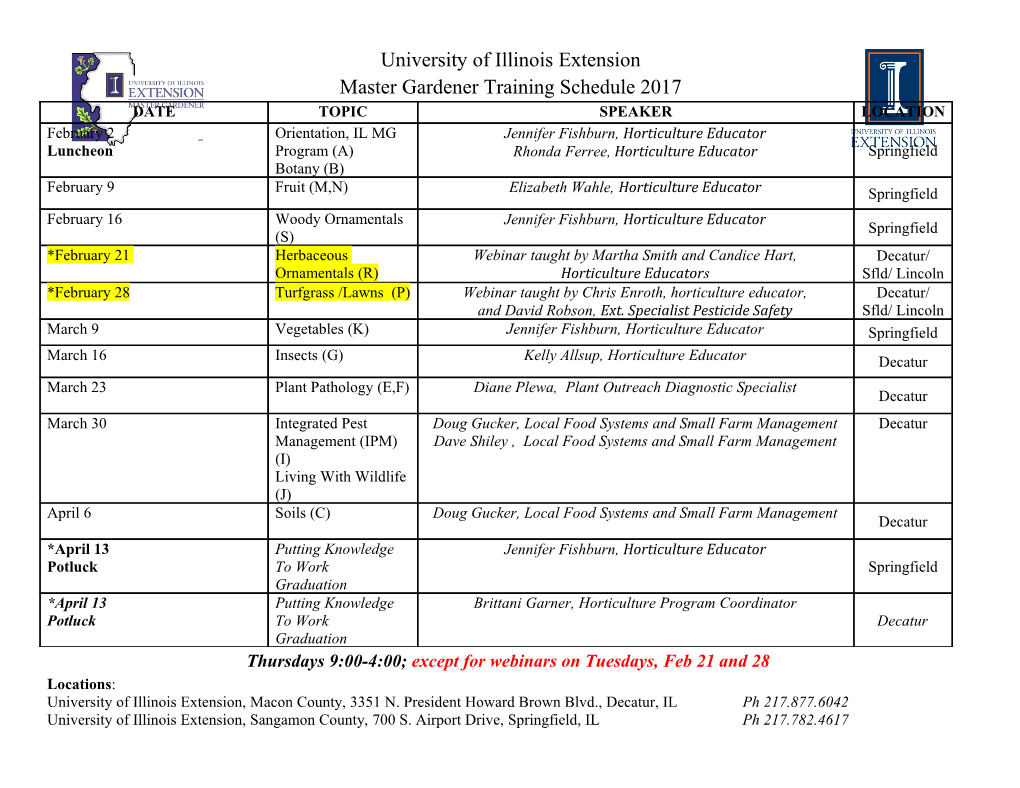
PH101 Lecture 12 ¬È¿Ä¹¿ÆÂ» ż ¨»·ÉÊ ¹Ê¿ÅÄ Lagrangian of system of particles ͆(ͥ%, ͥʖ%, ͨ) → Ê̋ Action integral ǹ ¨ ÇÀ,Çʖ À,Ê ºÊ Ê̊ A mechanical system will evolve in time in such that action integral is stationary Hamilton’s Principle of Least Action → Ê̋ Ê̋ Stationary ǹ ¨ ÇÀ,Çʖ À,Ê ºÊ î ǹ ¨ ÇÀ,Çʖ À, Ê ºÊ = ̉ Ê̊ Ê̊ Stationary condition of Action integral ͘ "͆ "͆ Lagrange’s equation from Variational − = 0 principle ͨ͘ "ͥʖ% "ͥ% symmetries and conservation laws Cyclic coordinates, symmetries and conservation laws Cyclic coordinate ( Corresponding generalized (canonical) ͥ%) momentum ( i conserved ͤ% = ) i,ʖĝ is independent of the particular ͆ cyclic coordinate ( . System is symmetric under translation ͥ%) has no effect on in generalized coordinate ͥ% → ͥ% + ͥ% ͆. ÇÀ Symmetry in generalized coordinate gives rise to a conserved canonical momentum. Example : For planetary motion 1 ͇́͡ ͆ = ͡ ͦʖ ͦ + ͦͦʖ ͦ + is independent of rotation angle (cyclic2 coordinate), the systemͦ has rotational symmetry ͆ [symmetric under translation in , the system remains the same after change in . → + ) As is cyclic, corresponding generalized (canonical) momentum ͦ =Constant; ͤW = ͦ͡ ʖ which is nothing but angular momentum. Conclusion : Conservation of angular momentum is related to rotational symmetry of the system. Translational symmetry and homogeneity of space Homogeneity of space : Space is such that in a particular direction, all the points are equivalent. Z Homogeneous in X-direction Potential at → every point along the line ͆ ͬ, ͭ, ͮ = ͆(ͬɑ, ͭ, ͮ) Î̊ Î̎ Y Homogeneous along X-direction is invariant under → ͆ translation along X-direction conserved X → ͤ3 Homogeneous along Y-direction is invariant under → ͆ translation along Y-direction conserved. → ͤ4 Homogeneous along Z-direction is invariant under → ͆ translation along Z-direction conserved → ͤ5 Rotational symmetry and isotropy of space Isotropy of space : Different directions around a point are all equivalent (at the same distance from that point). Thus → ɑ ɑ , function of ͆ ͦ, , , = ͆(ͦ, , , ) ͦ only. Isotropy of space Rotational symmetry of ≡ the system in both , and All the directions are equivalent Potential energy in different directions (at the same distance from a particular point) must be same, as all directions are equivalent. Thus L , and . , is invariant under rotation. ͦ ,͙͙͙ͤͨ͘͘͢͢͢͝ ͚ͣ ͆ and and are conserved. ͤe ͤW ͤ Symmetry and conservation laws Homogeneity of space Conservation of linear momentum (Translational symmetry ) Isotropy of space Conservation of angular momentum (Rotational symmetry) Homogeneity in time? ɑ ͆ ͥ%, ͥʖ%, ͨ = ͆ ͥ%, ͥʖ%, ͨ Only possible if does not have ͆ explicit time dependence Homogeneity in time (“translation” in time) Conservation of energy If does not explicitly depend on time, then energy of the system is ͆ conserved, provided potential energy is velocity independent. Homogeneity in time leads to energy conservation: Proof ͆ = ͆(ͥͥ, … ͥ), ͥʖͥ, … . ͥʖ), ͨ) Using the chain rule of partial differentiation ͆͘ "͆ "͆ "͆ = ȕ ͥʗ% + ȕ ͥʖ% + ͨ͘ "ͥʖ% "ͥ% "ͨ % % Using Lagrange’s ͆͘ "͆ ͘ "͆ "͆ = ȕ ͥʗ% + ȕ ͥʖ% + eqn. ͨ͘ "ͥʖ% ͨ͘ "ͥʖ% "ͨ % % ͘ "͆ "͆ − = 0 ͆͘ ͘ "͆ "͆ ͨ͘ "ͥʖ% "ͥ% = ȕ ͥʖ% + ͨ͘ ͨ͘ "ͥʖ% "ͨ % ͘ "͆ "͆ [1] ȕ ͥʖ% − ͆ + = 0 ͨ͘ "ͥʖ% "ͨ % If does not have explicit time dependence ͆ "͆ "͆ ȕ ͥʖ% − ͆ = ͕̽ͣͧͨͨ͢͢ ͝, ͙ = 0 "ͥʖ% 2 "ͨ % "͆ ȕ ͥʖ% = 2͎ "ͥʖ% % If does not depend on generalized velocity, i ; i i as ͐ = 0 = ͆ = ͎ − ͐ i,ʖ ĝ i,ʖĝ i,ʖĝ If does not explicitly depend on time ( i and V is velocity independent, ͆ i/ = 0) i ∑% ͥʖ% − ͆ = ͕̽ͣͧͨͨ͢͢ i,ʖ ĝ For a single free particle ͥ ͦ ͦ ͦ ͎ = ͡ ͬʖ + ͭʖ + ͮʖ ͦ "͎ ȕ ͥʖ% = ͡ ͬʖͬʖ + ͭʖͭʖ + ͮʖͮʖ = 2͎ "ͥʖ% % The relationship is true for General case as well, Euler’s theorem : If is a homogeneous function of the degree of set ͚(ͬ$) ͢/# of variables , then Ą¼ . ͬ$ ∑À Îʖ À = ļ ĄÎʖ À K is a function of 2 nd degree of generalized inetic energy ͎ velocities ͥʖ% Proof continue… "͎ Now, "͎ ȕ ͥʖ% − ͆ = ͕̽ͣͧͨͨ͢͢ ȕ ͥʖ% = 2͎ "ͥʖ% "ͥʖ% % % 2͎−͆ = ͕͗ͣͧͨͨ͢͢ 2͎−͎+͐ =͕͗ͣͧͨͨ͢͢ ͎ + ͐ = ͕͗ͣͧͨͨ͢͢ Conclusion , ͎+͐ = ̿ = ͕͗ͣͧͨͨ͢͢ , ͚͝ ͆ ͙ͣͧ͘ ͣͨ͢ ͙͙ͬͤ͗ͨͭ͠͝͝͠ ͙͙ͤ͘͘͢ ͣ͢ ͙ͨ͝͡ ͕͘͢ ͙͕ͤͣͨͨ͢͝͠ ͧ͝ ͙ͪͣ͗ͨͭ͠͝ ͙͙ͤͨ͘͘͢͢͝ Total energy conserved if does not depend not explicitly depend on time Change in time does ͆ → not cause any change in the form of Homogeneity in time ͆ → Summary Principle of least action : Action I= Ê̋ is stationary ȄÊ̊ ¨ ÇÀ,Çʖ À, Ê ºÊ ͘ "͆ "͆ − = 0 ͨ͘ "ͥʖ% "ͥ% If L does not have explicit time dependence, i,e ͆ = ͆ ͥͥ, … ͥ), ͥʖͥ, … . ͥʖ) "͆ ȕ ͥʖ% − ͆ = ͕̽ͣͧͨͨ͢͢ "ͥʖ% % 3v ɑ stationary=> º Ą¢ Ą¢ =0 I = Ȅ3u ̀ ͬ, ͭ, ͭ ͬ͘ ºÎ ĄÏɑ − ĄÏ If F does not have explicit dependence on , i,e ɑ ͬ ̀ = ̀ ͭ, ͭ "̀ ͭɑ − ̀ = ͕̽ͣͧͨͨ͢͢ "ͭɑ Homogeneity in space Conservation of linear momentum Isotropy of space Conservation of angular momentum Homogeneity in time Conservation of energy Application of variation principle: Shortest path between two points on the surface of a sphere Shortest path is the path along the great circle connecting the two points Elementary length between two (ͧ͘) points in spherical polar coordinates ͧ͘ ͦ = ͦ͘ ͦ + ͦͦͦ͘ + ͦͦͧ͢͝ ͦ ͘ ͦ ºÉ On the surface of the sphere, ͦ = ͌ = ͕͗ͣͧͨͨ͢͢ ͦʖ = 0 ͧ͘ ͦ = ͌ͦͦ͘ + ͌ͦͧ͢͝ ͦ ͘ ͦ Shortest path between two points on the surface of a sphere Total length between two points 1&2 Mathematically difficult due to non-zero i ͦ ͦ iW ͍ = ǹ ͧ͘ = ǹ ͌ͦͦ͘ + ͌ͦͧ͢͝ ͦ ͘ ͦ ͥ ͥ You can also express as ͦ Wv ev ͦ ͦ ͘ ͘ ͍ = ͌ ǹ 1 + ͧ͢͝ ͘ ͍ = ͌ ǹ ͧ͢͝ ͦ + ͘ Wu ͘ eu ͘ ͦ ɑ ͘ ɑ ͘ ͦ = ̀ , , () = 1 + ͧ͢͝ ͦ = 1 + ͧ͢͝ ͦɑ ͘ ͘ Necessary condition for the integral (total time) to be extremum º Ą¢ Ą¢ =0 − ºò ĄĀɑ ĄĀ Shortest path between two points on the surface of a sphere ͦ ɑ ͦ ɑͦ Ą¢ Ą¢ ͧ͢͝ Ā ̀ = 1 + ͧ͢͝ = ̉ ɑ = ĄĀ ĄĀ ͦ 1 + ͧ͢͝ ͦ ɑ ͘ ͧ͢͝ ͦĀɑ ͧ͢͝ ͦĀɑ = ̉; = ¹ÅÄÉÊ·ÄÊ = Á ͘ ͦ ͦ 1 + ͧ͢͝ ͦ ɑ 1 + ͧ͢͝ ͦ ɑ v ͨ ɑͦ ͦ ͦ ɑͦ ; ɑ & . W ͧ͢͝ = ͟ 1 + ͧ͢͝ = ± ͥͯ&v. vW ͦ ͦ ɑ ͟ ͗ͧ͗ ͟ ͗ͧ͗ = ± = ± 1 − ͦ͟(1 + ͗ͣͨ ͦ) 1 − ͦ͟ − ͦ͗ͣͨ͟ ͦ) ͦ ɑ ±͟ ͗ͧ͗ = 1 − ͦ͟ ͦ͟ 1 − ͗ͣͨ ͦ 1 − ͦ͟ Shortest path between two points on the surface of a sphere ͦ ͗ͧ͗ ͘ Let ±& and ͘ = = v ͥ = 1 − ͦ͗ͣͨ ͦ ͥͯ& , ; , cot ͘ = Ȅ ͘ = Ȅ ͥͯ,v ͥͯ,v ͥ͘ = α ͗ͧ͗ ͦ ͘ ͯͥ ; = sin ͥ + ͥ = sin( − ) → ͙͕̓ͨ͛ͦͨͣ͢͢͝ ͕͗ͣͧͨͨ͢͢ ë ¹ÅÊò = ̳̩̮(Ā−ì)……..[̊] To understand the meaning of , multiply both sides by »ÇË·Ê¿ÅÄ ̊ ͌ ͌ ͗ͣͨ = ͌ sin − ͌ ͗ͣͧ = ͌ ͧ͢͝ ͧ͢͝ ͗ͣͧ − ͌ ͧ͢͝ ͗ͣͧ ͧ͢͝ ͮ = ͗ͣͧ ͭ−sin ͬ Equation of a plane É¿Äì Î−¹ÅÉì Ï−ëÐ = ̉ passing through origin Equation of a plane passing through ͬͤ, ͭͤ, ͮͤ ͕ ͬ − ͬͤ + ͖ ͭ − ͭͤ + ͗ ͮ − ͮͤ = 0 Shortest path between two points on the surface of a sphere ͧ͢͝ ͬ−͗ͣͧ ͭ− ͮ = 0 This plane which passes through the origin slices through the sphere in great circles Thus solution of Euler-Lagrange’s equation are great circle routes Shortest path between two points on the surface of a sphere must lie on this the great circle passing through those points . Questions?.
Details
-
File Typepdf
-
Upload Time-
-
Content LanguagesEnglish
-
Upload UserAnonymous/Not logged-in
-
File Pages17 Page
-
File Size-