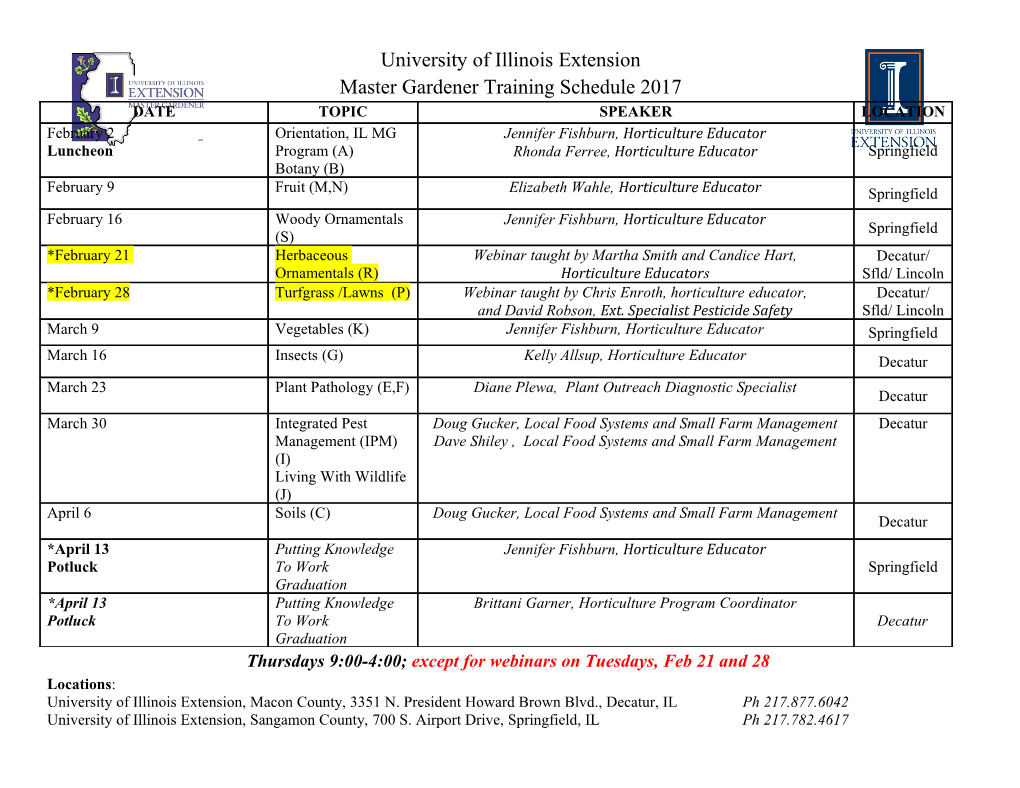
In Situ Probes in the Atmospheres of the Ice Giants Principal Author: Glenn Orton Jet Propulsion Laboratory, California Institute of Technology Pasadena, California 91109, USA ([email protected]) Co-Authors: David Atkinson1, Tibor Balint1, Mark Hofstadter1, Olivier Mousis2, Kunio Sayanagi3, Tom Spilker4 1JPL/Caltech, USA, 2Aix-Marseille Univ., France, 3Hampton Univ. USA, 4Independent consultant, USA Jet Propulsion Laboratory, California Institute of Technology Pasadena, California 91109, USA Abstract This paper describes atmospheric entry and descent probe missions in the atmospheres of the ice giants, Uranus and Neptune, targeting a minimum depth at the 10-bar level. In situ exploration of ice giant atmospheres addresses two broad themes: i) the formation history of the ice-giant planets and their role in the evolution of the solar system, and ii) the structure, dynamics, and processes in their atmospheres. An ice giant probe descending under parachute can measure abundances of key atmospheric constituents, deep thermal structure, dynamics, and processes, with data returned to Earth by a Carrier Relay Spacecraft. An atmospheric probe is an essential element of a possible future NASA Ice Giant New Frontier or Flagship mission. 1. Introduction The gas giant planets, Jupiter and Saturn, and the ice giant planets, Uranus and Neptune, contain most of the non-solar mass of the solar system and have played a significant role in shaping solar system evolution [1], influencing its current structure and affecting delivery of volatiles to the inner (terrestrial) planets [2]. Understanding the formation, evolution, and ultimate location of the giant planets is key to understanding the formation and evolution of the solar system as a whole. To extend knowledge and add additional clarity to the role played by the giant planets in solar system formation and evolution requires in situ exploration of the gas and ice giant planets. In particular, noble gases reflect both the location and processes of giant planet formation and subsequent evolution. Additionally, processes in ice giant atmospheres differ significantly from processes in the atmospheres of the gas giant planets. A key example is the predominance of retrograde rather than prograde winds at low latitudes, and the structure, location, and properties of condensate clouds in the significantly colder atmospheres found in the outer solar system. Although remote sensing is capable of making many important measurements of the composition of and processes within planetary atmospheres, remote sensing has limited capabilities to observe the deeper atmosphere beneath the clouds [3]. Atmospheric measurements beyond the reach of remote sensing require entry and descent probes to determine key atmospheric constituents including the abundance of noble gases and isotopes as well as the structure of and processes within the atmosphere beneath the cloud tops. Measurements of noble gas abundances and the ratio of key isotopes must be made in situ by a probe carrying a mass spectrometer, possibly including a tunable laser spectrometer and a dedicated helium abundance detector. Since noble gases are expected to be well-mixed, abundance measurements can be made by a relatively shallow probe in the upper atmosphere. Measurements of cloud condensates and condensables requires a vertical profile by a probe descending into the deeper atmosphere, down to 10 bars of pressure or more. Atmospheric processes including dynamics requires the descent probe to carry an atmospheric structure instrument with sensors for pressure and temperature, electrical properties, as well as an atmospheric acoustic properties to measure speed of sound from which the atmospheric ortho-para H2 ratio can be found. Other atmospheric processes including the net radiative flux transfer profile of the atmosphere and cloud location, composition, structure, and aerosol properties such as size, number density, and shape requires additional in situ instrumentation. Two themes define the science motivation and instrument requirements for in situ exploration of ice giant atmospheres: i) the formation history of the ice giant planets and the role of the ice giants in the evolution of the solar system, and ii) the structure, dynamics, and processes in ice giant atmospheres (Table 1). The details of these goals can be found in our companion white paper [4]. 1 Table 1. Notional Ice Giant Probe Science Traceability Matrix Science Measurement Example Comments/Notes Science Goals Objectives Requirements Instruments He/H : ±2% Well-mixed H S, H O, Determine well- 2 2 2 Ne/H, Ar/H, Kr/H, S, O isotopes, NH Understand the mixed atmos- 3 Xe/H: ±10% requires deep probe. formation of the pheric composi- C/H, N/H, S/H, P/H: RSE Atmospheric Ice Giant tion including ±10% MS/TLS absorption for profile Planets and their abundances 15N/14N, D/H: ±5% RSE/AtmAbs of microwave absor- role in the (relative to H) of 3He/4He: ±3% bers (NH and H S); evolution of the He, noble gases, 3 2 Ne, Ar, Kr, Xe isotopes Galileo probe HAD solar system C, N, S, O, and & 18O/17O/16O, 12C/13C: He measurement: key isotopes ±1% ±2% Measure the alti- tude profile of Pressure: ±1%, ASI temperature & Temperature: ±1 K pressure Required depth ³10 Measure atmos- bars Profile of atmospheric pheric dynamics dynamics: RSE/DWE including winds & ±5 m/s waves/tides Measure speed of Ortho-para H2 from Understand sound in atmo- Acoustic acoustic anemometer atmospheric sphere to deter- Speed of Sound: ±1% Anemometer, (speed of sound): circulation, the mine hydrogen ASI, MS/TLS molecular mass, processes by ortho/para ratio temperature required which energy is Determine abun- transferred out- CO, PH , AsH , GeH : dances of key dis- 3 3 4 MS/TLS wards from the ±10% interior, and the equilibrium species structure of the Particle optical proper- Characterize ver- cloud layers in ties, size distributions, tical structure, the Ice Giants number and mass composition, and Nephelometer densities, opacity, properties of cloud shapes, and & haze particles. composition Determine net Up & down visible flux: atmospheric ~.4-5 µm up & down Solar uncertain by radiative energy IR flux: 4-50 µm; Net Flux 2%; Protosolar balance; altitude λ/Δλ∼ 0.1-100 Radiometer ~larger --> ±5% profile of thermal ΔFlux ∼0.5Wm−2: requirement IR & solar visible Accuracy ±1% ASI: Atmospheric Structure Instrument; RSE: Radio Science Experiment; AtmAbs: Atmospheric Absorption; DWE: Doppler Wind Experiment; MS: Mass Spectrometer; TLS: Tunable Laser Spectrometer; HAD: Helium Abundance Detector 2 2. Probe Mission Implementation To measure the overall composition, thermal structure, and dynamics of planetary atmospheres requires in situ measurements by a probe carrying a Mass Spectrometer, possibly including a tunable laser spectrometer, an acoustic anemometer to measure the ortho-para H2 ratio from the speed of sound in the atmosphere, a helium abundance detector, and an Atmospheric Structure Instrument to measure the altitude profile of atmospheric temperature vs. pressure. Additional possible instruments include a Nephelometer to characterize cloud structure and aerosol properties, and a Net Flux Radiometer to measure atmospheric radiative energy structure. The probe would target a minimum depth of 10 bars, about 5 scale heights beneath the tropopause with a descent speed largely determined by the instrument capabilities and required sampling periods. Although the probe power, structure, thermal control, and telecommunications will likely allow operations beyond 10 bars, a balance must be found between the total science data volume requirements to achieve the high-priority mission goals, the capability of the probe telecommunications system to transmit the entire science, engineering, and housekeeping data set (including entry accelerometry and pre-entry/entry calibrations) within the descent telecommunications time window, and the probe descent profile allowing the probe to reach 10 bars. An ice giant atmospheric probe with data returned to Earth by an orbiting or flyby Carrier Relay Spacecraft (CRSC) could be carried as an element of a dedicated ice giant planet system exploration mission. For delivery to the proper entry interface point, the CRSC with probe attached is placed on a planetary entry trajectory, and is reoriented for release of the probe. Prior to release, the probe would be configured for coast, entry, and atmospheric descent. Following probe release, a deflect maneuver is performed to place the CRSC on the proper trajectory to receive the probe descent telemetry. The probe is awakened by the coast timer for sequential power-on, warm-up, and health checks prior to arrival at the entry location. The only data collected during entry would be from the entry accelerometers and possibly instrumentation in the heat shield, including sensors to measure heat shield entry ablation. The end of entry is triggered by the accelerometers initiating the parachute deployment sequence, release of the aeroshell, and the initiation of atmospheric descent- science operations. Parachute descent begins near the tropopause by deploying a drogue parachute which pulls off the probe Figure 1. Galileo Jupiter probe entry and descent aft cover which then extracts and profile. (Credit: NASA) deploys the main descent parachute. Once under parachute, the probe heatshield is released and the transmit-only descent telecommunications link from the probe to the CRSC is initiated. Probe science collection and relay transmission strategy would be
Details
-
File Typepdf
-
Upload Time-
-
Content LanguagesEnglish
-
Upload UserAnonymous/Not logged-in
-
File Pages8 Page
-
File Size-