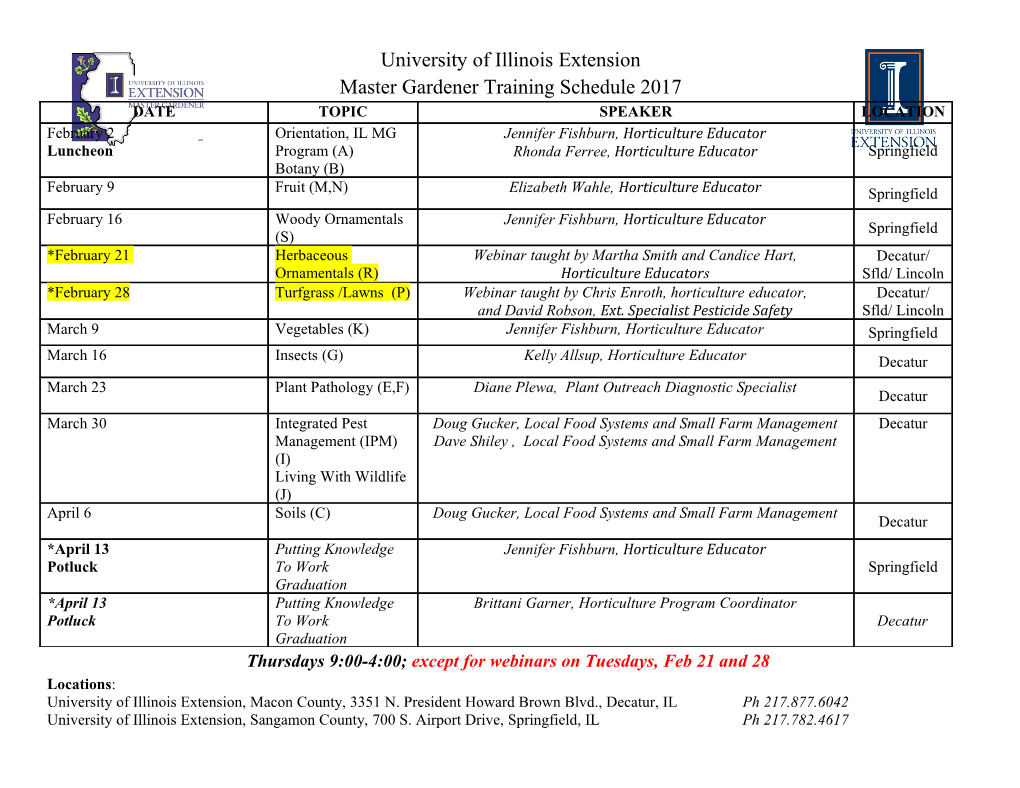
Published: 22 July 2010 © 2010 Medicine Reports Ltd Clinical applications of breath testing Kelly M Paschke1, Alquam Mashir1 and Raed A Dweik1,2* Addresses: 1Department of Pathobiology/Lerner Research Institute, Cleveland Clinic, Cleveland, OH 44195, USA; 2Department of Pulmonary and Critical Care Medicine/Respiratory Institute, Cleveland Clinic, Cleveland, OH 44195, USA * Corresponding author: Raed A Dweik ([email protected]) F1000 Medicine Reports 2010, 2:56 (doi:10.3410/M2-56) The electronic version of this article is the complete one and can be found at: http://f1000.com/reports/medicine/content/2/56 Abstract Breath testing has the potential to benefit the medical field as a cost-effective, non-invasive diagnostic tool for diseases of the lung and beyond. With growing evidence of clinical worth, standardization of methods, and new sensor and detection technologies the stage is set for breath testing to gain considerable attention and wider application in upcoming years. Introduction and context gases, in the breath [8]. Improved technologies such as With each breath exhaled thousands of molecules are selected-ion flow-tube MS (SIFT-MS), multi-capillary expelled, providing a window into the physiological column ion mobility MS (MCC-IMS), and proton transfer state of the body. The utilization of breath as a medical reaction MS (PTR-MS) have provided real time, precise test has been reported for centuries as demonstrated by identification of trace gases in human breath in the parts Hippocrates in his description of fetor oris and fetor per trillion range [9-11]. On the other hand, unlike hepaticus in his treatise on breath aroma and disease [1]. traditional quantitative breath analysis, the electronic nose Even in modern times clinicians have noted distinct is essentially trained to recognize odor patterns using an changes in the breath odor of patients with specific array of gas sensors. The electronic nose has shown diseases such as diabetes, renal failure, and hepatic accuracy in the detection of lung cancer, pneumonia, and diseases [2-4]. However, it was Linus Pauling’s milestone asthma with specificities and sensitivities ranging from 74- discovery of 250 unique substances present in exhaled 98%, as well as in the discrimination between diseases breath that offered promising insight into breath testing such as chronic obstructive pulmonary disease and asthma [5]. Since this discovery, breath analysis has rapidly [12-15]. Table 1 provides a selected list of the growing evolved as a new frontier in medical testing for disease number of technologies being applied to breath testing. states in the lung and beyond [1]. Breath analysis is now used clinically to monitor asthma, diagnose transplant More recent technological advancements in breath analy- organ rejection, diagnose Helicobacter pylori infection, sis have moved beyond measuring volatiles in the gas detect blood alcohol concentration, and monitor breath phase into measurement of semivolatiles and compounds gases during anesthesia, mechanical ventilation, and dissolved in aerosolized droplets in exhaled breath respiration, among numerous other applications [1,6,7]. condensate (EBC) and in exhaled breath vapor (EBV). Aerosolized droplets in EBC can be captured by a variety of Recent advances methods and analyzed for a wide range of biomarkers, Breath analysis may offer a relatively inexpensive, rapid, such as metabolic end products, proteins, cytokines, and and non-invasive method for detecting a variety of chemokines, with expanding possibilities [16,17]. With diseases. With recent advancements in mass spectrometry 3000 volatile compounds identifiable using EBC and (MS) and gas chromatography MS (GC-MS), it is possible twice the volatile metabolite concentration compared to to identify thousands of unique substances, such as traditional breath gas analysis, this application has the volatile organic compounds (VOCs) and elemental potential to provide superior information about Page 1 of 6 (page number not for citation purposes) F1000 Medicine Reports 2010, 2:56 http://f1000.com/reports/medicine/content/2/56 Table 1. Current breath-based test technologies Spectrometry Gas chromatography Other Mid-infrared absorption spectroscopy Automated thermal desorption gas chromatography Chemiluminescense mass spectrometry (ATD-GC-MS) Multi pass cell-laser absorption spectroscopy Gas chromatography flame ionization Electrochemical cell sensor technology detection (GC-FID) Tunable diode laser absorption spectroscopy (TDLAS) Gas chromatrography mass spectrometry (GC-MS) Gas sensor array technology Cavity ring-down spectroscopy (CRDS) Photoionization detection gas chromatography Nanosensor technology Cavity leak-out spectroscopy (CALOS) Solid phase microextraction gas chromatography mass Infrared and para-magnetic sensors spectrometry (SPME-GC-MS) Cavity enhanced optical frequency comb spectroscopy Gas chromatography time of flight mass spectrometry Calorimetry (GC-TOF-MS) Integrated cavity output spectroscopy (ICOS) Gas chromatography differential mobility spectrometry Sol-gel sensor technology (GC-DMS) Laser magnetic resonance spectroscopy (LMRS) Gas chromatography ion mobility mass spectrometry Laser based sensor technology (GC-IMS-MS) Laser photoacoustic spectroscopy Gas chromatography/Fourier transform ion cyclotron resonance mass spectrometry (GC/FT-ICR-MS) Faraday-LMRS Gas-liquid partition chromatography (GLPC) Selected ion flow tube mass spectrometry (SIFT-MS) Gas chromatography electrolyzer-powered flame ionization detector (GC-EFID) Proton transfer reaction mass spectrometry (PTR-MS) Faraday modulation spectroscopy Ion trap (2D) and (3D) mass spectrometry Time of flight mass spectrometry (TOF-MS) Isotope ratio mass spectrometry (IR-MS) Multi-capillary column ion mobility mass spectrometry (MCC-IMS) High sensitivity (hs)-PTR-MS Matrix assisted laser desorption/ionization time of flight mass spectrometry (MALDI-TOF-MS) Proton transfer reaction time of flight mass spectrometry (PTR-TOF-MS) breathprints of healthy and disease states [8,18]. EBV monitoring have served as a platform for technological sampling has also yielded promising results as a new growth in clinical breath testing applications. In parti- breath sampling method. EBV sampling pre-concentrates cular, earlier devices, such as those providing the breath samples using a solid-phase microextraction fiber detection of blood alcohol concentration, H. pylori inserted into a modified RTube™, a common device also infection, lactose intolerance, and airway monitoring used in EBC sampling. This procedure provides the by end-tidal carbon dioxide, have demonstrated clinical potential advantages of faster breath sampling and benefits as well as diagnostic success in clinical breath analysis, increased portability, minimal user training, use testing. Table 2 provides a selected list of the breath- in contaminated environments, and no requirement for a based tests currently approved by the FDA. power source. EBV sampling may yield additional compounds not detected in EBC and may provide greater One recent landmark in clinical breath testing occurred sensitivity as a sampling method, expanding the spectrum in 2003 when the FDA approved the first device that of breath sampling [19]. measures the fraction of exhaled nitric oxide (FENO) for asthma monitoring. The desktop NIOX® (currently Implications for clinical practice NIOX® FLEX) was followed by a handheld NIOX® The science of breath analysis is rapidly expanding, the MINO device (both by Aerocrine, Inc., Solna, Sweden) technology is improving, and several new applications that received FDA clearance in 2008. Advantages have been developed or are under commercial develop- provided by FENO monitoring devices include its non- ment. A major breakthrough over the past decade has invasive nature, ease of repeat measurements, and use in been the increase in breath-based tests approved by the adult and child populations with severe airflow obstruc- US Food and Drug Administration (FDA). Devices tion where other techniques would be difficult or measuring common breath gases: oxygen, nitrogen, impossible to perform [20]. FDA approval of these water vapor, and carbon dioxide in patient respiratory devices has largely been attributed to the standardization Page 2 of 6 (page number not for citation purposes) F1000 Medicine Reports Table 2. Breath-based tests approved by the US Food and Drug Administration [33] Molecule detected Disease/condition Trade name of analysis instrument Technology Manufacturer FDA approval date CO2, O2, N2O Respiration Consolidated-Nier model 21-201 Dual inlet system gas isotope Consolidated Electrodynamics Before 28 May 1976 isotope ratio mass spectrometer ratio mass spectrometer Corporation, Inc., Pasadena, CA, USA ® CO2 Respiration Tidal Wave Carbon Dioxide Sensor technology Novametrix Medical Systems, Inc., 20 November 1996 Monitor, Model 610 Wallingford, CT, USA H2 Lactose malabsorption Micro H2 Sensor technology MICRO DIRECT, Inc., Auburn, ME USA 24 January 1997 13 18 15 C, O, CO2, N, N2, Respiration ABCA-NT System Continuous flow gas isotope Europa Scientific, Ltd. Concord, MA USA 16 December 1997 2010, NO2 ratio mass spectrometer CO2, O2, N2O and Respiration, anesthesia Datex-Ohmeda Compact Airway Infrared sensor, paramagnetic Datex-Ohmeda, Inc., Tewksbury, MA, 23 August 2000 anesthetic agents Module M-CAiOVX and M-COVX sensor USA 2 13 12 ® :56 http://f1000.com/reports/medicine/content/2/56 CO2/ CO2 H. pylori UBiT -IR3000 Infrared Spectrometry Infrared
Details
-
File Typepdf
-
Upload Time-
-
Content LanguagesEnglish
-
Upload UserAnonymous/Not logged-in
-
File Pages6 Page
-
File Size-