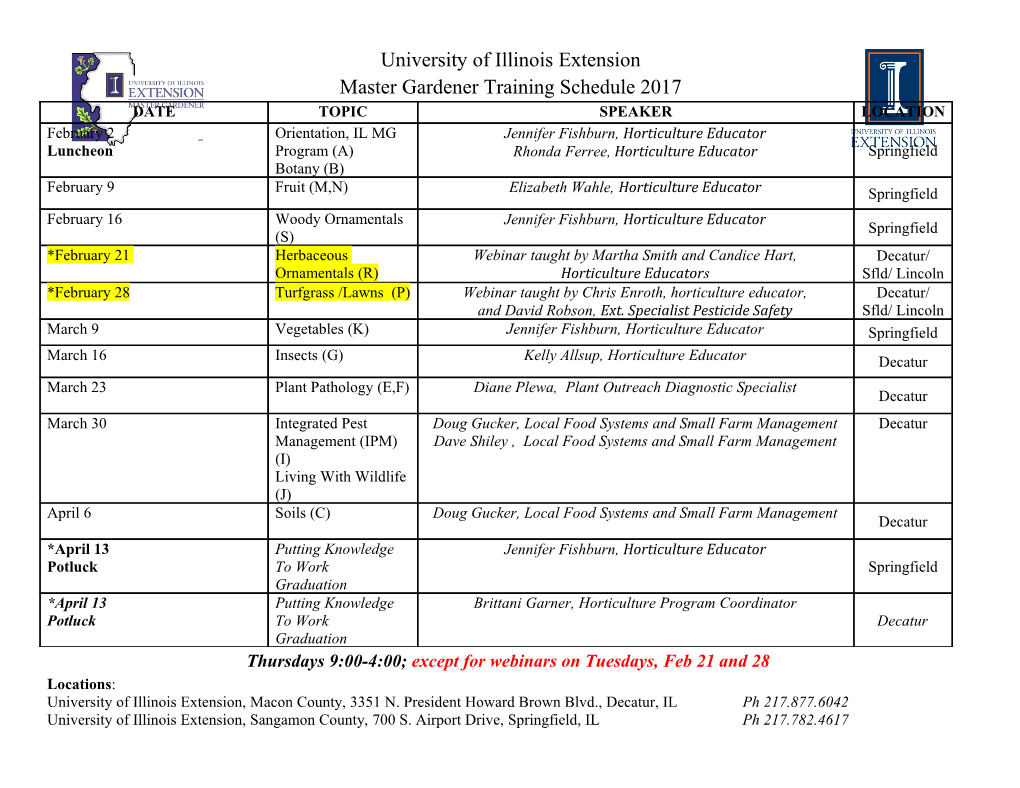
Article Cite This: J. Am. Chem. Soc. 2019, 141, 14878−14888 pubs.acs.org/JACS Strongly Lewis Acidic Metal−Organic Frameworks for Continuous Flow Catalysis † § † § † † ‡ † ‡ Pengfei Ji, , Xuanyu Feng, , Pau Oliveres, Zhe Li, , Akiko Murakami, Cheng Wang, † and Wenbin Lin*, † Department of Chemistry, The University of Chicago, 929 East 57th Street, Chicago, Illinois 60637, United States ‡ College of Chemistry and Chemical Engineering, iCHEM, State Key Laboratory of Physical Chemistry of Solid Surface, Xiamen University, Xiamen 361005, China *S Supporting Information ABSTRACT: The synthesis of highly acidic metal−organic frameworks (MOFs) has attracted significant research interest in recent years. We report here the design of a strongly Lewis acidic MOF, ZrOTf-BTC, through two-step transformation of MOF-808 (Zr-BTC) secondary building units (SBUs). Zr-BTC was first treated with 1 M hydrochloric acid solution to afford ZrOH-BTC by replacing each bridging formate group with a pair of hydroxide and water groups. The resultant ZrOH-BTC fl was further treated with trimethylsilyl tri ate (Me3SiOTf) to afford ZrOTf-BTC by taking advantage of the oxophilicity of the Me3Si group. Electron paramagnetic resonance spectra of Zr-bound superoxide and fluorescence spectra of Zr-bound N- methylacridone provided a quantitative measurement of Lewis acidity of ZrOTf-BTC with an energy splitting (ΔE) of 0.99 eV π * π * between the x and y orbitals, which is competitive to the homogeneous benchmark Sc(OTf)3. ZrOTf-BTC was shown to be a highly active solid Lewis acid catalyst for a broad range of important organic transformations under mild conditions, including Diels−Alder reaction, epoxide ring-opening reaction, Friedel−Crafts acylation, and alkene hydroalkoxylation reaction. The MOF catalyst outperformed Sc(OTf)3 in terms of both catalytic activity and catalyst lifetime. Moreover, we developed a ffi fl ZrOTf-BTC@SiO2 composite as an e cient solid Lewis acid catalyst for continuous ow catalysis. The Zr centers in ZrOTf- BTC@SiO2 feature identical coordination environment to ZrOTf-BTC based on spectroscopic evidence. ZrOTf-BTC@SiO2 displayed exceptionally high turnover numbers (TONs) of 1700 for Diels−Alder reaction, 2700 for epoxide ring-opening reaction, and 326 for Friedel−Crafts acylation under flow conditions. We have thus created strongly Lewis acidic sites in MOFs fl fl via tri ation and constructed the MOF@SiO2 composite for continuous ow catalysis of important organic transformations. ■ INTRODUCTION solubility in nonpolar organic solvents, are sensitive to Lewis acids efficiently catalyze many different types of organic moisture, and have short lifetimes during catalysis. In order See https://pubs.acs.org/sharingguidelines for options on how to legitimately share published articles. to overcome these drawbacks, researchers have devoted Downloaded via DALIAN INST OF CHEMICAL PHYSICS on November 21, 2019 at 12:05:37 (UTC). reactions by withdrawing electron density from functional − fi ff groups to make them susceptible to nucleophilic attacks.1 3 In signi cant e orts to developing heterogeneous Lewis acid Diels−Alder reactions, for example, coordination of a catalysts such as zeolites, metal oxides and resins over the past dienophile to a Lewis acid significantly decreases its LUMO few decades. Solid acid catalysts can be readily separated from 4 − reaction mixtures for reuse and are compatible with flow energy to facilitate its reaction with a diene. Catalytic Diels 9−11 Alder reactions allow for efficient synthesis of functionalized catalysis, but they have moderate Lewis acidity, low active site density, and nonuniform active sites.12,13 cyclic compounds that havefoundwidespreaduseas − pharmaceutical ingredients, artificial flavors, fragrances, and Metal organic frameworks (MOFs) have recently emerged agrochemicals.5 Lewis acids are also used to catalyze the as a novel porous material platform for designing homoge- neously inaccessible catalysts by taking advantage of their formation of epoxides and aromatic ketones and regioselective − regular framework structures and site isolation effects.14 19 In ring opening of epoxides to yield alcohols. ff Early Lewis acid catalysts were typically based on main particular, metal centers in MOF nodes have a orded group or early transition metal halides. Lewis acidity of these outstanding single-site solid catalysts with unique electronic metals increases when the halides are replaced with more properties and steric environments that are not accessible via electron-deficient and less coordinating anions, such as triflate − fl − 6−8 ( OTf) or bis(tri uoromethylsulfonyl)imide ( NTf2). Received: July 23, 2019 However, these Lewis acidic metal salts tend to have low Published: September 4, 2019 © 2019 American Chemical Society 14878 DOI: 10.1021/jacs.9b07891 J. Am. Chem. Soc. 2019, 141, 14878−14888 Journal of the American Chemical Society Article conventional homogeneous chemistry or heterogenization M aqueous HCl at 100 °C for 18 h to replace the formate approaches. Although MOF nodes have been used as Lewis groups with six pairs of Zr-coordinated hydroxide and water acidic sites to catalyze organic transformations, such as groups to afford ZrOH-BTC with the inorganic node 20 − 21 22,23 μ μ cyclization reactions, C H iodinations, hydrolysis, composition of Zr6( 3-O)4( 3-OH)4(RCO2)6[(OH)(OH2)]6. and dehydrations,24 using activated substrates, Lewis acidity ZrOH-BTC was then treated with trimethylsilyl triflate fi ° ff of these metal sites is signi cantly lower than the (Me3SiOTf) in benzene at 80 C for 8 h to a ord ZrOTf- 25 homogeneous benchmark Sc(OTf)3. We have previously BTC. As the oxophilic Me3Si group forms stronger bond with − − developed two quantitative methods for measuring Lewis the OH moiety than with the OTf moiety, Me3SiOTf readily acidity of MOF active sites through electron paramagnetic removes OH− from ZrOH-BTC to generate ZrOTf-BTC with μ μ resonance (EPR) spectroscopy of MOF-bound superoxide the node composition of Zr6( 3-O)4( 3-OH)4(RCO2)6[OTf]6. •− fl (O2 )or uorescence spectroscopy of MOF-bound N- The Me3SiOH byproduct generated through this activation 21 methylacridone (NMA). Quantitative measurements of reacted with excess Me3SiOTf to form (Me3Si)2O. The fi Lewis acidity of MOFs can potentially guide the discovery of amount of (Me3Si)2O was quanti ed to be 1.92 equiv w.r.t. highly Lewis acidic MOF catalysts for efficient organic Zr by 1H NMR, agreeing well with the proposed activation synthesis through continuous flow catalysis.21 process (Figure S3, Supporting Information). Several examples of Zr MOFs have recently been shown to − Thermogravimetric analysis (TGA) of ZrOTf-BTC showed possess relatively strong acidity.20,22,23,26 29 Notably, Yaghi a weight loss of 63.8% in the 220−800 °C range (Figure S2, ̈ and co-workers developed MOF-808-SO4 as a strong Bronsted Supporting Information), consistent with the expected weight μ μ acid catalyst for alkene dimerization. The unsaturated Zr6 loss of 62.8% for the conversion of Zr6( 3-O)4( 3- fi ff nodes of MOF-808 were modi ed by sulfuric acid to display OH)4(BTC)2(OTf)6 to (ZrO2)12. Powder X-ray di raction strong Brönsted acidity (Figure 1).30 By utilizing a distinct (PXRD) studies showed that ZrOTf-BTC remains crystalline and maintains the structure of ZrOH-BTC (Figure 2b). Transmission electron microscope (TEM) images of ZrOTf- BTC displayed highly crystalline octahedral particles of ∼200 nm in dimensions. (Figure 2c) The porosity of ZrOTf-BTC fi − was con rmed by N2 sorption isotherms, with a Brunauer Emmett−Teller (BET) surface area of 779 m2/g. (Figure 2d) Pore size analysis by nonlinear density functional-theory (NL- DFT) showed a uniform pore at ∼16 Å that is attributable to the large hexagonal cages of the MOF. The pore size of ZrOTf- BTC is smaller than that of ZrOH-BTC (21 Å) due to the presence of six large triflate groups per SBU (Figure 2e). Figure 1. Chemical structures of reported acidic Zr MOFs and Computational and Spectroscopic Studies of Zr-OTf ZrOTf-BTC. Active Sites. We used a range of computational and spectroscopic techniques, including density functional theory (DFT), extended X-ray absorption fine-structure (EXAFS) and strategy, we aim to develop strongly Lewis acidic Zr MOF X-ray absorption near-edge spectroscopy (XANES), to catalysts that work through different and complementary elucidate the Zr coordination environment in ZrOTf-BTC. reaction mechanisms in this work.31,32 We synthesized Lewis The first question we attempted to address is whether triflate acidic ZrOTf-BTC MOF from Zr-BTC (MOF-808) through groups are incorporated into the MOF. We performed sulfur sequential postsynthetic formate removal and triflation of K-edge XANES analysis to prove the presence of triflate MOF nodes. ZrOTf-BTC is a highly active solid Lewis acid groups. The sulfur centers in ZrOTf-BTC displayed significant catalyst for a broad range of important organic transformations, edge-step, with the K-edge energy of at 2479.8 eV. This energy including Diels−Alder reaction, epoxide ring-opening reaction, is much higher than that of sodium thiosulfate (Na S O ) − 2 2 3 Friedel Crafts acylation, and alkene hydroalkoxylation reac- standard and matched well to the edge energy of Sc(OTf)3, tion. ZrOTf-BTC exhibits higher Lewis acidity than Sc(OTf)3 indicating the presence of triflate groups in ZrOTf-BTC and outperforms Sc(OTf)3 in these reactions with higher (Figure 3b). catalytic activity and longer catalyst lifetime. We further The next question we attempted to address is how triflate ff supported ZrOTf-BTC on SiO2 to a ord a composite material groups are coordinated to Zr centers. We envisioned two as an efficient solid Lewis acid catalyst for continuous flow possible modes of triflate coordination (Figure 3a): OTf μ catalysis. bridging two proximal Zr centers (the 2-model) or OTf chelation to a single Zr center (the η2-model).
Details
-
File Typepdf
-
Upload Time-
-
Content LanguagesEnglish
-
Upload UserAnonymous/Not logged-in
-
File Pages11 Page
-
File Size-