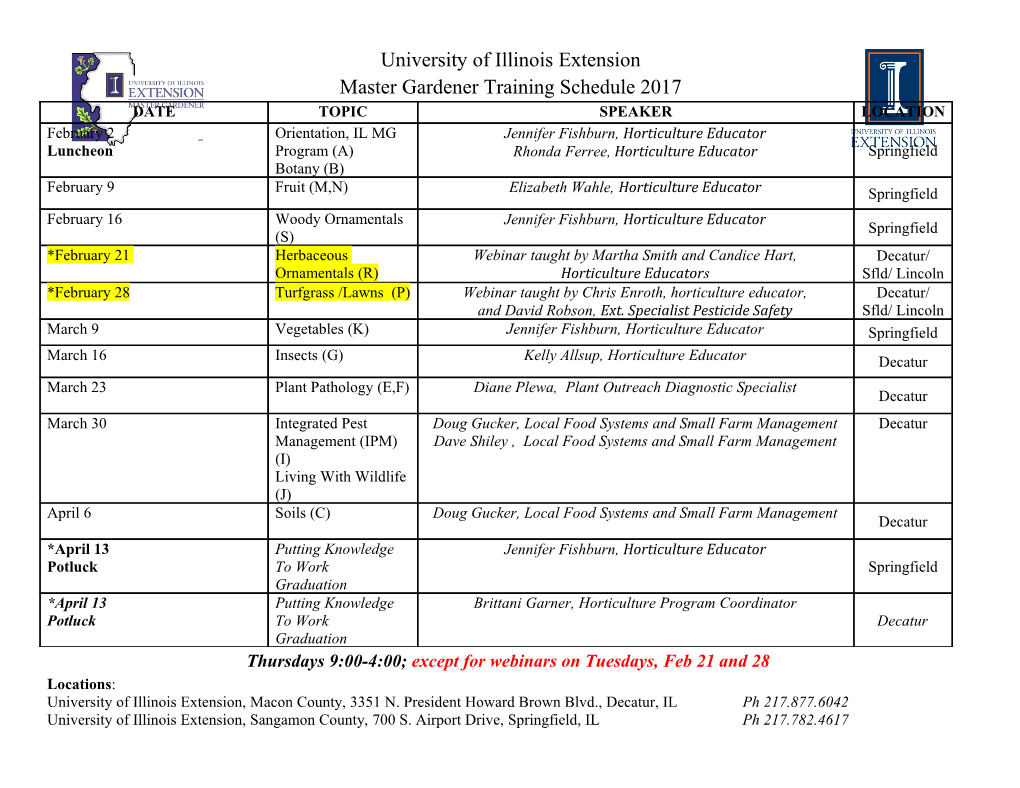
1 Design of a Thermal Neutron radiography Camera based on SARAF Accelerator Keren Lavie1,2, Yossi Eisen Ph.D1, Arnold Pernik Ph.D1 1Soreq NRC, Yavne, Israel, 2 Ben-gurion University, Beer Sheva, Israel 1. Introduction Neutron radiography is a powerful non-destructive imaging technique for the internal evaluation of materials or components. It involves attenuation of a neutron beam by an object to be radiographed, and the registration of the attenuation process as an image, on a film or a real time camera. Neutron radiography is an imaging technique which provides images similar to X-ray radiography. The difference between neutron and X-ray interaction mechanisms produce significantly different and often complimentary information. While X- ray attenuation is directly dependent on the atomic number, some light elements, such as hydrogen and boron have high thermal neutron attenuation coefficients, whereas some heavier elements, such as lead, have relatively smaller attenuation coefficients. Thermal neutron sources are obtained by moderating fast neutron sources. Fast neutron sources utilized for thermal neutron radiography (TNR) include nuclear reactors [1-8], proton and deuteron accelerators [7-11] and radioactive sources such as 252Cf [7-8]. Small research reactors of power in the range of 100 to 1000kW are typical of many reactor TNR facilities. Power of 250kW-50MW produces a neutron thermal flux of 1012-5x1014 n/cm2⋅sec. The types of accelerators available for fast neutrons are: cyclotrons, Van de Graaffs, sealed tube generators and R.F. Quadrapole Linacs. Paul Scherrer Institute (PSI) is operating the world’s strongest continuously running spallation neutron source (SINQ). It is driven by a 590 MeV proton beam generated by an isochronous cyclotron. A proton beam of 1mA produces a high energy spallation neutron yield of 3-6x1016 n/sec. The TNR facility at PSI (NEUTRA)[10] achieved at the image plane a thermal neutron flux between 3.5x106-2.5x107 n/cm2⋅sec. 252Cf, as an isotopic TNR neutron source, produces 2.40x109 n/sec per milligram 252Cf [7-8]. A thermal neutron source strength of about 3.4x108 n/cm2⋅sec was achieved using 50mg of 252Cf [7-8]. In this paper we describe calculations of a thermal neutron source and a TNR facility using the SARAF [12] superconducting linear accelerator. Fast neutrons are produced by the d+Be reaction. The energy of the deuterons is 40MeV and the beam current is 2mA. The yield of fast neutrons is 8⋅1014 n/sec and the flux of thermal neutrons at the center of a specially designed moderator is 1.7x1012 n/cm2⋅sec. The optimal moderator and the optimal collimator system for the TNR were obtained by Monte Carlo calculations using the MCNP-4b code [13]. 2. Soreq TNR neutron using a 5MW light water reactor The current thermal neutron radiography at Soreq NRC is based on a 5MW light water reactor. The thermal neutron source flux at the reactor core is 5x1013 n/cm2⋅sec and the flux of thermal neutrons entering the converging collimator is about 5x1012 n/cm2⋅sec. The camera includes a homogenous thermal neutron source, a convergent collimator, a beam defining collimator, a divergent collimator, an image plane and a thermal neutron beam catcher. The camera has the following characteristics: 2 (a) Neutron flux at the image plane is 6x105 n/cm2⋅sec. (b) The ratio (L/d) between the distance (L) [defining collimator- image plane] and the aperture diameter(d) is 250. (c) Cadmium ratio which is used to characterize the thermal beam purity is about 15. 3. Neutron radiography camera using SARAF The thermal neutron radiography camera utilizing both SARAF as the fast neutron source and specially designed moderator and collimator, is planned to improve the current camera characteristics. Simulation calculations were performed using MCNP 4b [13], and the optimal configuration of the moderator and the collimator were determined. Figure 1 displays the geometry of the camera including the moderator and the collimator. Figure 1: Camera configuration (not to scale) The thermal neutron camera is based on moderation of fast neutrons. The production of a high intensity fast neutrons is achieved by the 9Be(d,xn) reaction [14-15]. The beryllium target is a water cooled rotating disc. The 6mm thick beryllium target enables the absorption of the incident 40MeV deuterons. Neutron moderation is achieved by a D2O sphere surrounds the beryllium target at the region of interaction. The beryllium moderator-reflector is of thickness 20cm and the graphite moderator of thickness 10cm surrounds the beryllium. Thermal neutrons emitted at an angle 135o with respect to the incident beam direction, are passed through a converging collimator and a boral precollimator into a 2cm diameter boral defining aperture. The neutron beam is then diverged from the defining aperture towards the image plane of diameter 60 cm. The ratio (L/d) = 250, determines a distance L=500cm. In order to achieve a maximum and uniform thermal neutron flux, with minimum contribution of fast and epithermal neutrons and minimum contamination by gamma rays, a 7.6cm thick bismuth single crystal filter, cooled to liquid nitrogen temperature, was positioned before the boral defining collimator. In order to reduce fast and epithermal neutrons emerging out of the moderating sphere and reduce the contribution of thermal neutrons not originating from the D2O sphere, iron, borated polyethylene and cadmium 3 surround the thermal neutron beam line. Lead was added to absorb gamma rays, created by inelastic scattering and thermal neutron capture Monte-Carlo calculations for the optimum configuration show that the thermal neutron flux on the image plane is 6.8 x105 n/cm2⋅sec. The Cadmium ratio calculated in the image plane is about 11.2. Gamma flux is comparable to 180 mR/h. 4. Summary A design configuration of the moderator and the neutron collimator system that provides maximum thermal neutron intensity as well as high thermal to epithermal and fast neutron ratio at the image plane was determined by detailed Monte Carlo calculations. This collimator design yields similar characteristics to the current thermal neutron camera. The engineering design of the neutron moderator and the collimator system, based on the results of this study, is in progress. 5. References 1. F.C. de Beer, W.J.Strydom, “neutron radiography: facilities and applications at the SAFARI-1 research reactor in south Africa”, proceeding of sixth World Conference on NR, Osaka, Japan, May 1999, 101 2. Y. G. Jo, N. M. Abdurrahman, B. W. Wehring, "Design of a Neutron Radiography Collimator System in a Through Beam Port at TRIGA Reactor," Trans. Am. Nucl. Soc., 75, 113 (1996) 3. J. Altamirano et. al, “Development of a neutron radiography system at the TRIGA MARK III reactor of the nuclear center of Mexico”, proceeding of first World Conference on NR, San Diego, December 1981, 119-126 4. R.A. Lillie et. al, “design calculations for a 14MeV neutron collimator”, Nuclear Technology, vol. 43, May 1979, 373-381 5. J.S. Brenizer et. al, “Recent improvements to the Pennsylvania State University’s neutron radiography facility”, proceeding of sixth World Conference on NR, Osaka, Japan, May 1999. 6. T. Matsumoto, “Towards design of neutron beams for neutron radiography at the proposed MUSASHI reactor”, proceeding of sixth World Conference on NR, Osaka, Japan, May 1999, 159-165. 7. M.R. Hawkesworth and J. Walker, “Basic principles of thermal neutron radiography”, proceeding of first World Conference on NR, San Diego, December 1981, 5-21. 8. W. Whittemore “Neutron source and facilities –present status and future”, proceeding of sixth World Conference on NR, Osaka, Japan, May 1999, 3-9. 9. E. Lehmann, et. al., “Application of new radiation detection techniques at the Paul Scherrer Institute, especially at spallation neutron source”, Nucl. Instr. and Meth. 424 (1999) 158-164. 10. E. Lehmann, et. al, “Properties of the radiography facility NEUTRA and SINQ and its potential for use as European reference facility”, proceeding of sixth World Conference on NR, Osaka, Japan, May 1999, 151. 11. T.D.Hayward, et. al, “Alight tandem accelerator for neutron radiography”, Nucl. Instr. and Meth. B10/11 (1985) 1077-1081. 12. A. Nagler ea. al, “SARAF Annual Report 2002”, Soreq NRC report 3344, March 2003. 13. MCNP version 4b, Monte Carlo N-Particle Transport Code System, Los Alamos National Laboratory, Los Alamos, New Mexico,1997. 14. J.P. Meulders et al. ,”Neutron Yields from Targets Bombarded with Deuterons”, Phys. Med. Biol.(1975), vol 20, no. 2, page 235-243. 15. M.J. Saltmarsh, “Characteristics of an intense neutron source based on the d+Be reaction”, Nucl. Instr. and Meth. 145 (1977) 81-90. .
Details
-
File Typepdf
-
Upload Time-
-
Content LanguagesEnglish
-
Upload UserAnonymous/Not logged-in
-
File Pages3 Page
-
File Size-