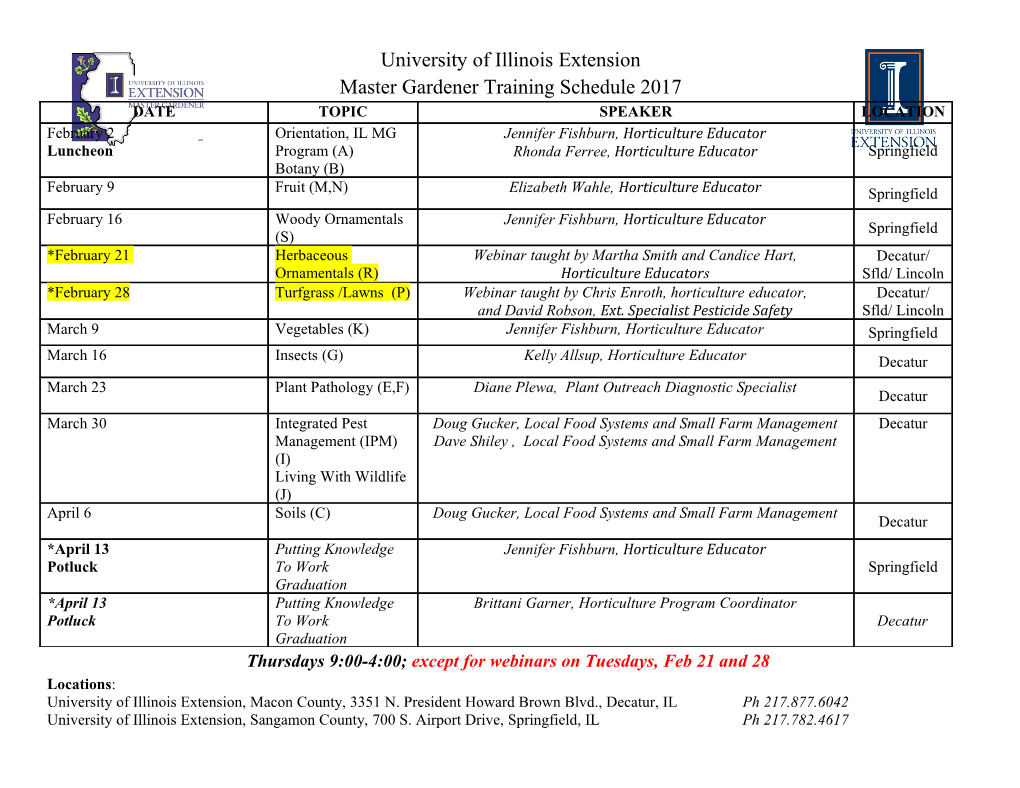
Life Out of Sequence Life Out of Sequence: A Data-Driven History of Bioinformatics Hallam Stevens The University of Chicago Press :: Chicago and London Hallam Stevens is assistant professor at Nanyang Technological University in Singapore. The University of Chicago Press, Chicago 60637 The University of Chicago Press, Ltd., London © 2013 by The University of Chicago All rights reserved. Published 2013. Printed in the United States of America 22 21 20 19 18 17 16 15 14 13 1 2 3 4 5 isbn-13: 978-0-226-08017-8 (cloth) isbn-13: 978-0-226-08020-8 (paper) isbn-13: 978-0-226-08034-5 (e-book) doi: 10.7208/chicago/9780226080345.001.0001 Library of Congress Cataloging-in-Publication Data Stevens, Hallam. Life out of sequence : a data-driven history of bioinformatics / Hallam Stevens. pages. cm. Includes bibliographical references and index. isbn 978-0-226-08017-8 (cloth : alk. paper) — isbn 978-0-226- 08020-8 (pbk. : alk. paper) — isbn 978-0-226-08034-5 (e-book) 1. Bioinformatics—History. I. Title. qh324.2.s726 2013 572′.330285—dc23 2013009937 This paper meets the requirements of ansi/niso z39.48-1992 (Permanence of Paper). For my parents If information is pattern, then noninformation should be the absence of pattern, that is, randomness. This commonsense expectation ran into unexpected com- plications when certain developments within information theory implied that information could be equated with randomness as well as pattern. Identifying information with both pattern and randomness proved to be a powerful para- dox, leading to the realization that in some instances, an infusion of noise into a system can cause it to reorganize at a higher level of complexity. Within such a system, pattern and randomness are bound together in a complex dialectic that makes them not so much opposites as complements or supplements to one an- other. Each helps to defi ne the other; each contributes to the fl ow ofinformation through the system. n. katherine hayles, How We Became Posthuman: Virtual Bodies in Cybernetics, Literature, and Informatics I put my faith in randomness, not order. j. craig venter, A Life Decoded: My Genome: My Life Contents Introduction 1 1 Building Computers 13 2 Making Knowledge 43 3 Organizing Space 71 4 Following Data 107 5 Ordering Objects 137 6 Seeing Genomes 171 Conclusion: The End of Bioinformatics 203 Acknowledgments 225 Archival Sources 229 Notes 231 Bibliography 259 Index 281 Introduction Will the domestication of high technology, which we have seen marching from triumph to triumph with the advent of personal computers and GPS receivers and digital cameras, soon be extended from physical technology to biotech- nology? I believe that the answer to this ques- tion is yes. Here I am bold enough to make a defi nite prediction. I predict that the domesti- cation of biotechnology will dominate our lives during the next fi fty years at least as much as the domestication of computers has dominated our lives during the previous fi fty years.1 Freeman Dyson wrote these words in 2007. Dyson was one of the physicists who laid the groundwork for the transformation of physics and physics-based technol- ogy in the second half of the twentieth century. He is not alone in suggesting that biology will be to the twenty-fi rst century what physics was to the twentieth. Over the last several decades, biology has already been transformed. The growing importance of bioinformatics—computers, data, and data management—signals that fundamental changes have taken place in how biologists work and how they build knowledge. INTRODUCTION 2 But the great success and the ultimate “domestication” of physics in the second half of the twentieth century came at a signifi cant price. The discipline was overhauled to an extent that would have made it hardly recognizable to practitioners of just a few decades earlier. Physics was transformed by the need for more money, more manpower, and more machines as well as by military involvement.2 Physicists struggled with their changing identity as their work became increasingly oriented to- ward the management of large interdisciplinary teams working at cen- tralized institutions. What it meant to do physics was fundamentally transformed over the middle decades of the century—practices, institu- tions, reward structures, career paths, education, and the core knowl- edge of the discipline were altered. This transition did not occur without a struggle. Some physicists— and some non-physicists—worried intensely about what the advent of Big Science would mean not only for their own professional futures, but also for the future of scientifi c knowledge. Although it was Donald Glaser’s invention of the bubble chamber that provided the model for the fi rst large-scale postwar physics laboratories, he was so disturbed by the new culture of physics that he quit the discipline entirely. Glaser longed for the days of the individual scientist working diligently at his lab bench and disliked the corporate, collective world that physics had become.3 In his valedictory address, President Dwight Eisenhower famously worried about the corrupting effects that large amounts of money would have on the purity of scientifi cally produced knowledge; the “military- industrial complex,” he fretted, posed an immediate threat to free ideas and intellectual curiosity: Today, the solitary inventor, tinkering in his shop, has been over- shadowed by task forces of scientists in laboratories and testing fi elds. In the same fashion, the free university, historically the fountainhead of free ideas and scientifi c discovery, has experi- enced a revolution in the conduct of research. Partly because of the huge costs involved, a government contract becomes virtu- ally a substitute for intellectual curiosity. For every old black- board there are now hundreds of new electronic computers.4 Alvin Weinberg, director of the sprawling Oak Ridge National Labora- tory, which had helped to build the fi rst atomic bombs, was also con- cerned about the effects of Big Science on both scientists and society. These effects were felt most strongly by the individual investigator: INTRODUCTION 3 The research professor in a simpler day concerned himself with the substance of his science, both in research and teaching. Now, through no fault of his own, he must concern himself with many other matters. To do his research, he has to manage, even in Little Science, fairly large sums of government money. He must write justifi cations for his grants; he must serve on commit- tees that recommend who should receive support, who should not; he must travel to Washington either to advise a govern- ment agency or to cajole a reluctant contract administrator. In short, the research professor must be an operator as well as a scientist.5 Such changes, Weinberg argued, led to reduced fl exibility in scientifi c practice, compromised its “intellectual discipline,” created increasing fragmentation between the specialized branches of science, and forced diffi cult decisions about what kinds of science to support. Weinberg par- ticularly criticized high-energy physics—the apotheosis of Big Science— for its failure to address problems of human welfare and technology and for its remoteness even from other branches of physics.6 Those who worked in less well funded subdisciplines, such as solid-state physics, wondered whether high-energy physics really deserved so much cash. In 1972, Philip W. Anderson argued that the “arrogance” of particle phys- ics was causing important domains of investigation to be under-studied and underfunded; solid-state physics presented an alternative to the re- ductionist approach of Big Science.7 Such arguments lay at the surface of a deeper pool of discomfort about what money, machines, and the military had done to physics. Big Science forced refl ection on the most appropriate ways to make physical knowledge.8 Biology, as it too becomes Big Science, is currently experiencing many of the same changes that revolutionized physics in the mid-twentieth century. Signifi cantly, many of these changes are being driven by the computerization of biological work and knowledge. Eisenhower’s image of blackboards being transformed into electronic computers reminds us that computers not only played a crucial role in transforming physics, but also became an important symbol of the new styles of working and knowing. Information technologies—computers, networks, robotics— are playing an even more central role in transforming the practices of biology in the early twenty-fi rst century. What did a biology laboratory look like before computers, Big Sci- ence, and genomics? The ethnographers Bruno Latour and Steve Woolgar gave a fi rsthand account of a neuroendocrinology lab from the 1970s. INTRODUCTION 4 They described workers preparing assays, attending to instruments, and recording data, as well as the fl ows of paper (mail, journal offprints, and typed manuscripts), animals, and chemicals through the lab.9 No doubt there are many laboratories that still more or less conform to this description: work is organized around benches, which are fi lled with reagents and samples, technicians and scientists communicate and col- laborate with one other primarily in face-to-face interactions around blackboards or whiteboards, and the most important things that come in and out of the laboratory are physical materials and printed papers. But by the mid-1980s, changes were afoot. In 1991, Walter Gilbert, one of the inventors of DNA sequencing, proclaimed the coming of a “paradigm shift”
Details
-
File Typepdf
-
Upload Time-
-
Content LanguagesEnglish
-
Upload UserAnonymous/Not logged-in
-
File Pages303 Page
-
File Size-