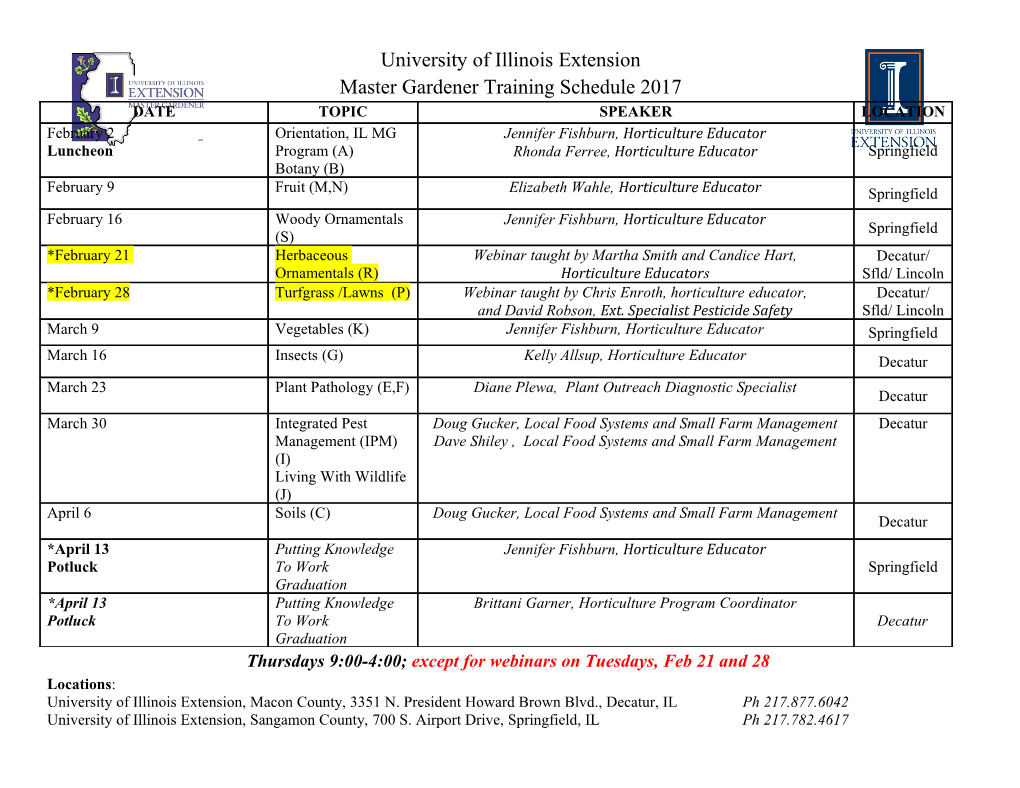
www.ierjournal.org International Engineering Research Journal (IERJ) Special Issue Page 1197-1201, June 2016, ISSN 2395-1621 Induction Hardening on Drive axle shaft and it’s FEA #1Aniket A. Deshmukh, #2Prof. D.H. Burande 1 PG student, Automotive Engineering, Mechanical Engineering Department, Savitribai Phule Pune University, Pune, Maharashtra, India. 2Associate professor, Mechanical Engineering Department, Savitribai Phule Pune University, Pune, Maharashtra, India. Abstract: This work deals with increasing strength of steel drive axle shafts by placing an extra bush support. It includes the modeling of shaft in CATIA. Drive shaft made up of MS material will be analyzed first. Stress and deformation will be the output of analysis. The meshing and boundary condition application will be carried using Hypermesh; Structural analysis of shaft will be carried out using ANSYS. Re-designing the shaft placing a bush for additional support in the length of shaft, and applying induction hardening process at the place of bush support for increasing the strength and reducing the deflection of the shaft. The design optimization also improves the performance of drive shaft. Keywords: Finite Element Analysis, Drive axle shaft, Induction hardening, Bush support. 1. INTRODUCTION Power is transmitted from engine to differential through propeller shaft, Axle shaft is connected between Hardening process is used for parts that wears while differential and wheel. It takes torque from engine. In this operating. During hardening core of the part does not get paper, drive axle shaft of Bolero automobile is thought of affected. Hardening increases wear resistance of a for the study. This axle shaft is semi-floating kind. component. Most surface treatments result in compressive Advantages of having semi-floating axle are that it’s the residual stresses at the surface that reduces the chance of simplest and most cost effective and they are widely used crack initiation and does not allow crack propagation at in automobiles. the case-core interface. Induction heating is a particularly versatile heating method 2. LITERATURE SURVEY which will perform uniform surface hardening, localized Torsion strength of axle shaft is determined by surface hardening, through hardening, and tempering of considering detailed spline and the material gradation due hardened pieces. Heating is accomplished by placing a to the hardness profile. Six different spline geometries and steel ferrous part in the magnetic field force generated by seven different hardness profiles including non-hardened high-frequency electrical energy passing through a degree and through-hardened shafts have been considered of inductance, usually a water-cooled copper coil. The (Barsoum et al, 2014). depth of heating produced by induction is connected to the Study investigates the influence of continuous cast section frequency of the electrical energy, power input, time, part size on the mechanical performance of induction hardened coupling and quench delay. The higher the frequency, the components created from steel bars. Hot rolled round bar of thinner or shallow the heating. Therefore, deeper case diameters 37–44mm is normalized and machined. This bar is depths and even through hardening are created by using then tested for its life prediction through fatigue analysis (Robert Crydermana et al, 2011) low range of frequencies. Considering this electrical involve the phenomena of hysteresis and eddy currents. Fractured axle shafts made of induction-hardened steel is Because secondary and radiant heat is eliminated, the studied. Several microstructure and hardness tests like optical process is suited to in-line production. Some of the microscopes, field emission gun scanning electron advantages of induction hardening are rapid process, microscopy (FEG-SEM), energy dispersive spectroscopy energy efficiency, less distortion, and small footprints. (EDS) is done. Microstructural analysis and micro- hardness Care must be exercised when holes, slots, or other special profile showed that the shaft was improperly heat treated geometric features will be induction hardened, which will resulting in a brittle case, from where crack initiates from the concentrate eddy currents and lead to warming of coils and case in a brittle mode in contrast to ductile mode within the cracking. core. The analysis suggests that the fracture gets initiated from the martensitic www.ierjournal.org International Engineering Research Journal (IERJ) Special Issue Page 1197-1201, June 2016, ISSN 2395-1621 case as brittle mode due to improper heat treatment process (high hardness) (Souvik Das et al, 2015) From the results of simulation it was found that the total length of the axle grows about 2.3 mm after induction hardening, and the shaft shrinks about 4 μm radially. Induction heating affects the residual stress distribution ( Dr. Lynn Ferguson et al, 1998). Fatigue life was predicted by making a two-layer model based on case and core material fatigue properties. Fatigue life prediction of case hardened steel specimen by considering variable loading conditions is done (Fengjie Yin et al, 2013) Two sets of case hardened steel of 25-mm-diameter were studied for their magnetic properties with the aim of developing various methods to validate different case depths nondestructively (Chongxue Zhang et al, 2012) Fig 1: Drive axle shaft while hand measurement The axle is said to have failed before rollover if the calculable force for failure is equal to or less than forces that acts on the axle during events leading to the rollover (C. Kendall Clarke, Don Halimunanda, 2010) The axle shaft forging/extrusions are machined and shaped to a close net shape condition and then induction hardened and quenched in water to 40 HRC with and effective case depth (ECD) of 0.120 to 0.250 in. (3 to 6.3 mm) in the barrel of the shaft. The results of this work show that effective tempering of induction hardened axle shafts is dependent on actual heat transfer rates and component temperature instead of on time at a given ambient furnace temperature (Thomas Neumann, Kenneth Pickett, 2009) 3. Problem statement: When rear axle fails it will cause a rollover under certain Fig 2: Actual image of a Bolero drive axle shaft during conditions. These conditions usually occur in SUVs as a measurement result of their higher center of gravity. This work aims at increasing the strength of the shaft. 4. Generation of CAD model & Analysis on existing drive axle shaft The conventional model used in Bolero vehicles is used. Dimensions are taken through reverse engg i.e through hand calculations. CAD model then is made by the commands in CATIA of Pad, pocket, fillet, and geometrical selections in part design module. Parametric generation of drawings will help to get the dimensions useful in forces calculations in static and dynamic loading conditions on axle shaft. Figure 1, 2 shows actual image of axle shaft while taking hand measurement. Figure 3 & 4 shows CAD and meshed model drawn in CATIAV5 and Hypermesh respectively. Fig 3: CAD model of axle shaft in CATIAV5 Meshing details are: Type of mesh: Tetra-hedral No. of Nodes: 10039 No. of Elements: 52656 Proper quality criteria have been maintained while meshing of axle shaft. Important quality check for 3D mesh is tetcollapse. www.ierjournal.org International Engineering Research Journal (IERJ) Special Issue Page 1197-1201, June 2016, ISSN 2395-1621 Table 1: Material properties for Mild Steel Property Value 5 Young’s Modulus, E 2.1x10 MPa Poisson’s Ratio ,ν 0.3 Density, ρ 7850 kg/m3 Yield Stress, σyield 290 MPa Ultimate Tensile Stress, 390 MPa σuts 4.3 Post-processing: After preparing deck for static analysis, model is exported Fig 4: Meshing of axle shaft in Hypermesh into ANSYS format i.e. .cdb and solution is run. Following are the results for Von-mises stresses and 4.1 Boundary Condition and calculation: deformation in an existing drive axle shaft. Specifications of a car engine: Displacement = 2523 cc Max power = 46.3 KW Max. torque = 195 Nm Transfer gear ration = 3.78 Torque Calculation Velocity, V = 120 Km/h = 2000 m/min Tire Diameter, D = 27.6 in = 703 mm = 0.703 m Vehicle RPM = Speed of Vehicle / Circumference of tire Nv = V/ (π*D) = 2000/ (π*0.703) Nv = 905.57 rpm Drive shaft rpm, N = Nv*gear Ratio = 905.57*3.78 N = 3423.05 rpm Power, P = 2*π*N*T/60 3 Fig 5: von-mises stress for axle shaft 46.3*10 = 2*π*2053.8*T/60 Stress value for axle shaft is 166.945 N/mm2 which is T = 129.16 Nm well below the critical value. Hence, design is safe. Abuse torque by considering FOS= 3.5 T The maximum displacement is coming out to be 2.5mm. applied = 129.16 * 3.5 = 452.07 Nm We see that the maximum stress is observed at wheel hub side. So there is maximum possibility that the axle shaft will fail at this point. To prevent this Bush is provided as a support. 5. Iterations: 5.1 Analysis with one support: Fig5: Constraints and forces applied on model in Hypermesh Boundry Conditions: Shaft is constrained at the one end and 452.07 Nm torque is applied on the other end as shown in figure 5. 4.2 Material Properties for MS (Mild Steel): Conventional material used in axle shaft is MS whose material Fig 6: Axle shaft with one bush properties are listed below: Above figure shows the same boundary conditions applied After applying boundary conditions, material properties as applied to existing shaft. Bush is placed where there are assigned. Material properties necessary to give input to were more chance of axle failure. the software is Poisson’s ratio, young’s modulus, and density. www.ierjournal.org International Engineering Research Journal (IERJ) Special Issue Page 1197-1201, June 2016, ISSN 2395-1621 Following are the results displayed for stress and Stress value for axle shaft is 167.18 N/mm2 which is deformation: below the critical value.
Details
-
File Typepdf
-
Upload Time-
-
Content LanguagesEnglish
-
Upload UserAnonymous/Not logged-in
-
File Pages5 Page
-
File Size-