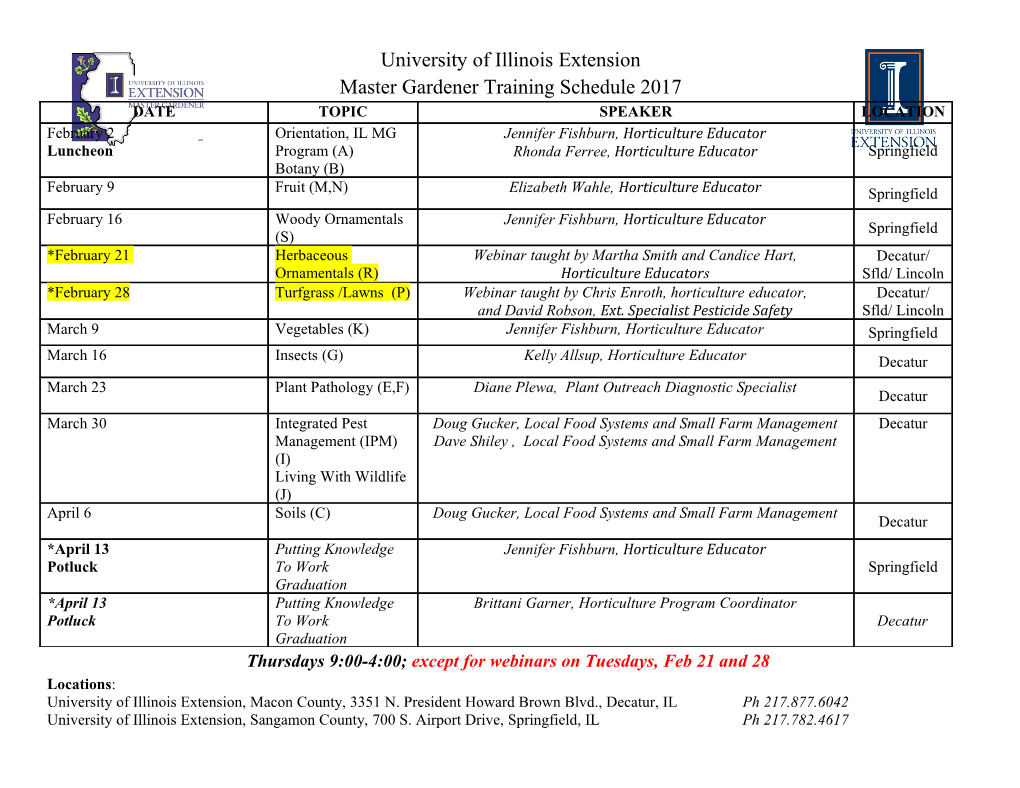
PNL-3249 UC-78 3 3679 00054 4074 MASS FLOWS FOR LMFBRs FUELED WITH VARIOUS TYPES OF PLUTONIUM AND FERTILE MATERIAL U. P. Jenquin December 1979 Prepared for the U.S. Department of Energy under Contract EY-76-C-06-1830 Pacific Northwest Laboratory Richland, Washington 99352 CONTENTS ACKNOWLEDGMENT • v INTRODUCTION 1 SUMMARY 3 REACTOR DESCRIPTION . 4 CALCULATION MODEL 6 CROSS SECTIONS 6 REACTIVITY AND BURNUP 7 RESUL TS 9 ENRICHMENT 9 MASS FLOW • 11 BREEDING RATIO . 13 CONCLUS IONS 15 REFERENCES 17 APPENDIX A - MASS-FLOW DATA A.l iii ACKNOWLEDGMENT Appreciation is expressed to D. R. Marr of the Hanford Engineering Devel­ opment Laboratory (HEDL) for making the computer codes and cross sections available. v INTRODUCTION Under the current policy of operating light water reactors on a once­ through cycle until the breeder reactor is introduced, breeder reactors must obtain their plutonium initially from the spent U0 2 fuel. This report pre­ sents results of neutronics analyses performed to determine fueling require­ ments and mass flows for a typical 1200 megawatt electric (MWe) liquid metal fast breeder reactor (LMFBR). This information could be used in future anal­ yses of requirements for particular generating scenarios. In performing the analyses, three plutonium compositions were considered to fuel the reactor. The composition depended on the amount of irradiation either a U02 or Pu02-Th0 2 fuel had received in a light water reactor (LWR). Uranium and thorium fertile materials were considered. Calculations were per­ formed to determine the cross sections, reactivity, and burnup for each fuel­ fertile material configuration. Based on these calculations, the mass flows, plutonium enrichment requirements, and breeding ratios for each configuration were determined. This study was prompted by the potential symbiotic relationship between LWRs and LMFBRs. For instance, the fuel produced by the LMFBR will have a very high fissile content. Thus, it may be more useful to use this bred fuel in LWRs and continue to fuel LMFBRs with plutonium discharged from LWRs. When using thorium fertile material in the LMFBR, the discharged uranium will have a very high 233 U content. When using uranium fertile material in the LMFBR blankets, the discharged plutonium will have a very high 239 pu content. However, uranium fertile material in the LMFBR core will produce plutonium containing a large percentage of 240 pu • This plutonium is used more effi­ ciently in the LMFBR than the LWR. As 233 U is recycled in LWRs, it may be desirable to recy~le LWR-discharged plutonium back in the LWR if the LMFBRs cannot use all of the plutonium. This is the main reason LMFBR calculations were done with plutonium obtained from a Pu-Th fueled LWR. This study does not address the use of the bred fuel in LWRs. This study was funded under the Fuels Refabrication and Development Program. It parallels a study done by HEDL(1) and reported in Nuclear Technology (February 1979). The HEDL report was focused upon the effect of 1 various blanket materials as well as use of both 233 U and plutonium to fuel the LMFBR. The HEDL study was directed toward the objectives under the Non­ proliferation Alternative Systems Assessment Program (NASAP). In contrast to this study, the HEDL study included only one plutonium composition--that of U0 2 fuel discharged at 30,000 MWd/MTM and evaluation of thorium data. This study utilized more recent thorium data in which the absorption cross sections are significantly different from those used by HEDL. 2 SUMMARY Plutonium enrichment requirements, mass flows, and breeding ratios are presented for a 1,200 MWe LMFBR using plutonium with one of three isotopic compositions and either uranium or thorium fertile materials in the blankets and the core. The mass-flow data for the LMFBR were obtained through calculation of cross sections, flux, reactivity, burn up and breeding ratios for each fuel­ fertile material configuration. The amount of plutonium required to fuel an LMFBR is most sensitive to the isotopic composition of the plutonium and the type of fertile material used in the core. When the fissile fraction of the plutonium decreases, the total plutonium requirement increases but the fissile plutonium requirement decreases. The highest breeding ratios are obtained using the plutonium with the lowest fissile fraction. A change from uranium to thorium in the axial or radial blankets has little effect on enrichment requirements. However, changing the fertile material in the core from uranium to thorium increases the enrichment requirement by 25%. The LMFBR mass-flow data generated in this study may be used to analyze symbiotic relationships with LWRs. It may be more beneficial to use the bred 233 u and 239 pu to fuel LWRs because of the high fissile fraction of the product. The plutonium generated by LWRs has a lower fissile fraction; hence, it may be more beneficial to use it to fuel LMFBRs. 3 REACTOR DESCRIPTION The study was performed for a reactor based on the General Electric 1200 MWe advanced oxide design.(2) The reactor operates at full power for 315 days per year. Three plutonium compositions were considered as fuel for the reactor and uranium and thorium were considered as fertile materials. The core and axial blanket parameters for the reference reactor are given in Table 1. The radial blanket parameters are also given in Table 1. A radial reflector, made of Inconel, was assumed to be 13.2 in. thick. The design did not include an axial reflector. The core and axial blanket are replaced every two years while the radial blanket is replaced every five years. TABLE 1. Design Parameters Core and Axial Radi al Blanket Blanket Electric Power (MWe)(a) 1200 Thermal Power (MWt)(a) 3736 Core Radius (in.) 65.3 Core Height (in.) 46.8 Blanket Thickness ( in. ) 13.0 13.6 Number of Fuel Assemblies 380 331 Number of Control Assemblies 20 Fuel Pin Diameter (in.) 0.286 0.519 Pins Per Assembly 271 61 Cladding Thickness (in.) 0.012 0.015 Fuel Smear Density (% TD)(b} 88 91 Core Composition (Vol. %) Fue 1 41.43 55.32 Structure 14.96 16.97 Sodium 43.61 27.71 Average Coo 1ant Temperature (oC) 470 315 ( a) 315 Full Power Days/Year (b) Percent of Theoretical Density 4 The structural material is assumed to be 316 stainless steel with elemen­ tal percentages of 68.5, 17, 12, and 2.5 for iron, chromium, nickel, and molyb­ denum, respectively. The fertile uranium used in the core and blankets was assumed to be 0.3% depleted, i.e. 99.7% 238 U and 0.3% 235 U. The p1utonium enrichment required to maintain a critical reactor from startup through equilibrium was determined for three isotopic compositions of plutonium. The isotopics are identified in Table 2. The material referred to as LEU @ 30 is the plutonium discharged from an LWR after 30 GWd/MTM exposure, where the fuel initially consisted of low-enriched U0 2. The LEU @ 50 pluto­ nium is similar1y described but discharged after 50 GWd/MTM exposure. The p1utonium referred to as Pu-Th @ 55 is the discharge from an LWR fueled with plutonium and thorium and irradiated to 55 GWd/MTM. For this latter case, the plutonium charged to the LWR in the form of a Th02-Pu02 fresh fue1 was type LEU @ 30. TABLE 2. Plutonium Compositions(3) ComEosition 2 atom % IsotoEe LEU @ 30 LEU @ 50 Pu-Th @ 55 238 pu 1.28 3.02 4.75 239 pu 58.83 50.96 20.73 240 pu 22.84 24.19 35.62 241pu 12.94 14.69 24.41 242pu 4.11 7.14 14.49 The reactor composition will be identified by nomenc1ature such as Pu-U8/U8/Th. The first item in this namenclaturerefers to the enriching mate­ rial in the core. Throughout this study, Pu is the only material used. The second, third, and fourth items in the nomenclature refer to the fertile mate­ rials in the core, axial blanket, and radial blanket, respectively. These will be either depleted uranium (U8) or thorium (Th). 5 CALCULATIONAL MODEL The analytical approach taken in this study included generation of broad-group cross sections, which were then used in a reactivity and burnup calculation. Results of these calculations consisted of enrichment require­ ments, mass flows, and breeding ratios. The calculational methods are described below. The results of the calculations are presented in the next section. CROSS SECTIONS Four-group cross sections were generated with 1DX(4) by collapsing 42-group cross sections over both the core and blanket neutron spectra individ­ ually. The lOX code was used to perform a one-dimensional two-region multi­ group diffusion theory calculation to generate neutron spectra for collapsing the cross-section data. The core consists of a uniform fuel mixture at a typ­ ical plutonium enrichment with appropriate isotopics. The radial blanket mix­ ture was homogenized with appropriate volume fractions of fuel, structural, and coolant materials. Four-group cross sections were averaged over the core and over the radial blanket for several core enrichments using various pluto­ nium isotopics. The four groups are described in Table 3. The bulk of the 42-group cross sections were derived from ENDF/B-IV data by HEDL. For 232 Th , preliminary Version V data, which are ~ubstantially more accurate than Version IV data, were used. The half-life of 241pu was taken as 14.5 years. The results are insensitive to the half-lives of the other isotopes. TABLE 3. Energy Boundaries for Broad-Group Cross Sections GrouE No. Lethar9~ Width Ener9~ Boundaries! eV 1 2.5 8.209 x 105 to 1.0 x 10 7 2 2.0 1.111 x 105 to 8.209 x 105 3 2.0 1.503 x 10 4 to 1.111 x 10 5 4 0.0 to 1.503 x 104 6 REACTIVITY AND BURNUP Reactivity and burnup calculations were based on the 4-group cross sec­ tions generated with 20B.
Details
-
File Typepdf
-
Upload Time-
-
Content LanguagesEnglish
-
Upload UserAnonymous/Not logged-in
-
File Pages43 Page
-
File Size-