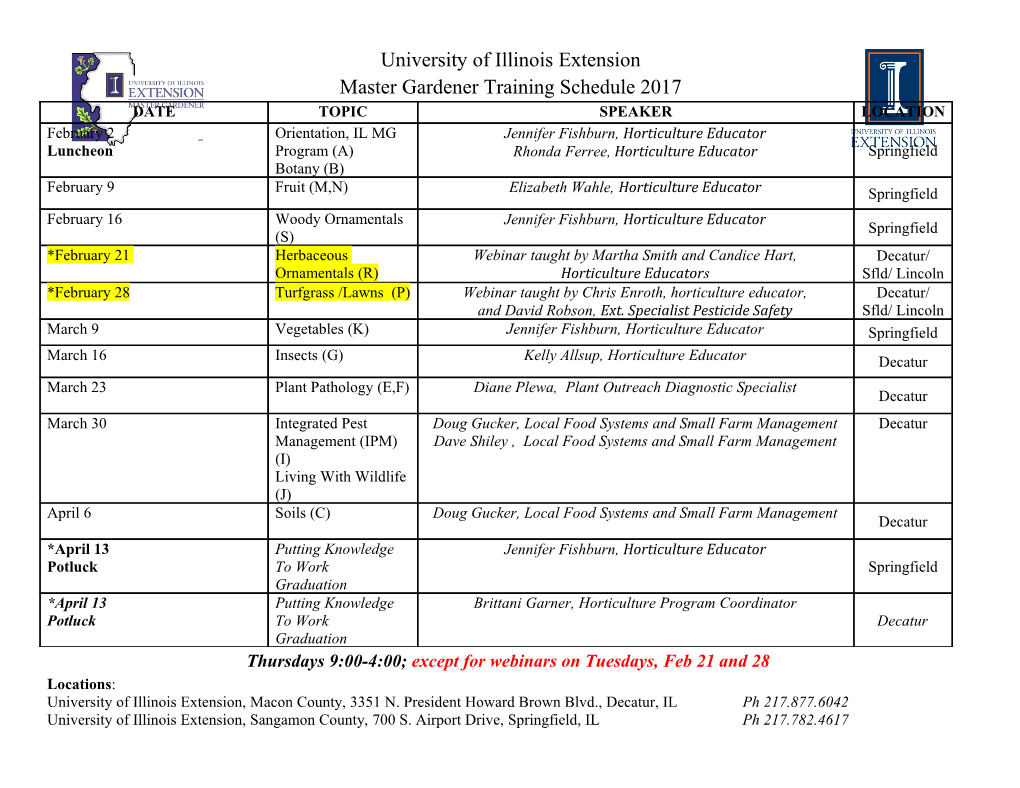
EUROPEAN ORGANIZATION FOR NUCLEAR RESEARCH Proposal to the ISOLDE and Neutron Time-of-Flight Committee Neutron capture cross sections of 70;72;73;74;76Ge at n TOF EAR-1 September 23, 2013 C. Lederer1, J. Andrzejewski2, M. Barbagallo3, E. Chiaveri4, C. Domingo-Pardo5, R. Dressler6, P. Ferreira7, I.F. Gon¸calves7, C. Guerrero4, F. Gunsing8, F. K¨appeler9, J. Neuhausen6, C. Massimi10, J. Perkowski2, R. Reifarth1, D. Schumann6, G. Tagliente3, J.L. Tain5, P. Vaz7, A. Wallner11, C. Weiß4;12, and the n TOF Collaboration. 1Goethe University Frankfurt, Germany 2UniwersytetL´odzki,Lodz, Poland 3INFN Bari, Italy 4CERN, Switzerland 5IFIC Valencia, Spain 6PSI, Switzerland 7Universidade T´ecnica de Lisboa, Lisboa, Portugal 8CEA Saclay, France 9Karlsruhe Institute of Technology, Germany 10INFN and University of Bologna, Italy 11Australian National University, Australia 12Technical University of Vienna, Austria Spokesperson: C. Lederer, [email protected] Technical coordinator: O. Aberle, [email protected] Abstract: We propose to measure the (n; γ) cross sections of the isotopes CERN-INTC-2013-021 / INTC-P-381 23/09/2013 70;72;73;74;76Ge. Neutron induced reactions on Ge are of importance for the astrophysical slow neutron capture process, which is responsible for forming about half of the overall elemental abundances heavier than Fe. The neutron capture cross section on Ge affects the abundances produced in this process for a number of heavier isotopes up to a mass number of A = 90. Additionally, neutron capture on Ge is of interest for low background experiments involving Ge detectors. Experimental cross section data presently available for Ge(n; γ) are scarce and cover only a fraction of the neutron energy range of interest. (n; γ) cross sections will be measured in the full energy range from 25 meV to about 200 keV at n TOF EAR-1. Requested protons: 12 × 1018 protons on target 1 1 Motivation Elements heavier than Fe are dominantly produced by neutron capture reactions in stars. About half of the elemental abundances are generated in the slow neutron capture process (s process) in stellar environments of neutron densities between 106 − 1012 cm−3. This implies that the nucleosynthesis path proceeds along the stability valley, since β-decays are usually faster than subsequent neutron captures on unstable species. The other half of elemental abundances is produced by the rapid neutron capture process (r process). This process is associated with very high neutron densities of > 1020 cm−3 and the reaction flow is driven towards the neutron rich side since neutron captures are faster than radioactive decays. The s process can be divided in two components called the main and the weak s process. The weak component of the s process involves s-process abundances between Fe and Zr and takes place in massive stars (M > 8 M ) during He Core burning, and later during C Shell burning. The main component, synthesizing nuclides between Zr and Bi happens in intermediate mass stars (1 < M < 5 M ) during their phase as a thermally pulsing Red Giant. The key nuclear physics inputs for studying the s process and calculating s-process abundances are stellar decay half-lives and stellar neutron capture cross sections, that is the cross section averaged over the stellar neutron spectrum (Maxwellian Averaged Cross Section, MACS). The MACS is defined as 2 1 Z 1 E p < σ >= 2 σ(E)E exp(− )dE (1) π (kBT ) 0 kBT where E is the neutron energy, kB is the Boltzmann constant and T is the temperature in the star. Since the s process takes place during different burning stages of stars, temperatures range from 0.1 to 1 GK, corresponding to kBT values of 8 − 90 keV. For determination of the MACSs, the excitation function needs to be known up to neutron energies of a few hundred keV. Particularly for the elements formed in the weak s process, stellar cross sections are required with an accuracy of at least 5% [1] for all involved nuclei since the change of only one cross section influences the abundances of successively heavier isotopes [2, 3]. Ge isotopes are produced in the weak s process. The s-process path in this region is shown schematically on the chart of nuclides in Figure 1. The isotope 70Ge, highlighted in purple, plays a special role. It is an "s-only" nuclide, which cannot be produced by the r process since it is shielded from β decays coming from the neutron rich side by its stable isobar 70Zn. s-only nuclides can be used to extract important s-process parameters, such as the average number of neutrons per Fe seed and the mean neutron exposure (time-integrated neutron flux) [4]. Neutron capture cross sections of germanium have a crucial influence on abundances of elements from germanium to yttrium. We investigated the sensitivity of s-process abundances to the stellar 72Ge(n; γ) cross section by increasing the MACS by a factor of 2 relative to the currently recommended cross section of the compilation KADoNiS-v3.0 [6]. The results obtained with the reaction network tool NETZ [5] are displayed in Figure 2 and show that abundances from germanium up to yttrium change by up to 50%. Capture cross sections of germanium isotopes are therefore crucial to understand the formation of successively heavier elements. 2 Figure 1: The s process in the region around Ge. The s-process path goes along the stability valley via neutron capture reactions (purple) and subsequent β decays (green and red). Additionally to its importance for stellar nucleosynthesis, neutron induced reactions on Figure 2: The effect of cross-section uncertainties on the s-process efficiency in massive stars illustrated at the example of 72Ge(n; γ) [5]. The plot shows the relative change of the iso- topic abundances when enhancing the stellar 72Ge(n; γ) cross section relative to the currently recommended value by a factor of 2. germanium play an important role in low background experiments, mostly due to the fact that Ge is used as detector material. This is the case e.g. for the Cryogenic Dark Matter Search (CDMS-II) experiment [8]. Another example is the The GERmanium Detector Array GERDA at Gran Sasso National Laboratory which uses the decay of 76Ge as probe to investigate neutrinoless double β decay. In both cases, neutron induced reactions yield to a signal, which is very hard to distinguish from the signal of interest [7, 8]. Thus, accurate neutron capture rates are crucial for treating the related (n; γ) background. Present experimental (n; γ) cross section data on Ge are scarce and often discrepant. For 72Ge and 73Ge, only one measurement above thermal neutron energies exists [9], covering 3 neutron energies up to 5 keV, while no data are available for the astrophysically important higher keV region. For 70Ge(n; γ), datasets extend up to about 200 keV neutron energy [9, 10, 11], but from 10 keV onwards there is no information on neutron resonances and only the unresolved cross section is given. The 74Ge(n; γ) and 76Ge(n; γ) cross sections have been measured by the time-of-flight technique up to 5 keV [9], for higher energies only activation data exist. We propose to measure the neutron capture cross sections of 70;72;73;74;76Ge at the n TOF facility, for the first time covering the full neutron energy range from 25 meV to 200 keV. 2 Experimental Setup and Beam Time Request We aim to perform the measurement at Experimental Area 1 (EAR-1) of the n TOF facility. The high neutron energy resolution combined with the high instantaneous neutron flux at n TOF will enable us to analyse most of the neutron capture resonances up to few hundred keV neutron energy. The prompt γ-radiation emitted after each neutron capture event will be detected using the C6D6 detection setup already installed at n TOF. These detectors are optimized for a very low sensitivity to scattered neutrons, which represent an important background component. The samples will consist of 2 grams of isotopically enriched oxide powder which will be pressed into a pellet of cylindrical form with a diameter of 2 cm and additionally sintered to increase their stability. We calculated 18 the expected count rate for the C6D6 detection setup for each Ge sample with 2 × 10 protons on the spallation target. For all isotopes we used the evaluated neutron capture cross sections of the library ENDF/B-VII [12]. The results for a resolution of 5000 bins per energy decade, which is required to perform a resonance shape analysis, are shown in Figures 3-7. Ge-70 5.23e-3 at/b, 5000 bins per decade 106 background level Counts in resonance 105 104 3 Counts / 2e18 protons 10 102 10 1 103 104 105 Neutron Energy (eV) Figure 3: Count rate estimates for 70Ge, using the sample specifiation as of Table 1. The integral number of counts in the individual resonances are shown as black points. The blue line indicates the level of the background. The blue line in all figures indicates the level of the expected background, which has been estimated from previous experiments. In some cases the background in the unresolved 4 Ge-72 5.23e-3 at/b, 5000 bins per decade 7 10 background level 106 Counts in resonance 105 104 3 Counts / 2e18 protons 10 102 10 1 103 104 105 Neutron Energy (eV) Figure 4: Count rate estimates for 72Ge, using the sample specifiation as of Table 1. 108 5.04e-3 at/b, 5000 bins per decade 107 background level Counts in resonance 106 105 104 Counts / 2e18 protons 103 102 10 1 103 104 105 Neutron Energy (eV) Figure 5: Count rate estimates for 73Ge, using the sample specifiation as of Table 1.
Details
-
File Typepdf
-
Upload Time-
-
Content LanguagesEnglish
-
Upload UserAnonymous/Not logged-in
-
File Pages8 Page
-
File Size-