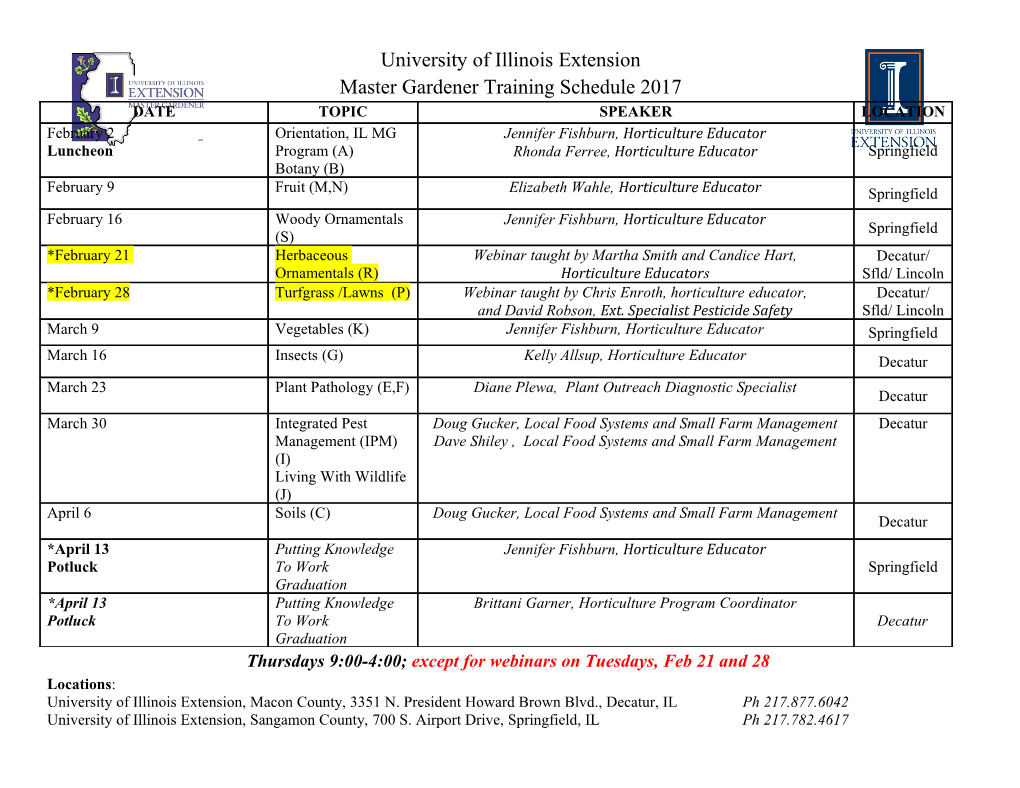
www.nature.com/scientificreports OPEN Benchtop micro‑mashing: high‑throughput, robust, experimental beer brewing Edward D. Kerr, Christopher H. Caboche, Peter Josh & Benjamin L. Schulz* Brewing science is undergoing a renaissance with the use of modern analytical chemistry and microbiology techniques. However, these modern analytical tools and techniques are not necessarily aligned with the scale and scope of brewing science. In particular, brewing processes can be time consuming, ingredient intensive, and require specialised technical equipment. These drawbacks compound with the need for appropriate numbers of replicates for adequately powered experimental design. Here, we describe a micro‑scale mash method that can be performed using a common laboratory benchtop shaker/incubator, allowing for high throughput mashing and easy sample replication for statistical analysis. Proteomic profles at both the protein and peptide levels were consistent between the 1 mL micro‑mash and a 23 L Braumeister mash, and both mash scales produced wort with equivalent fermentable sugar and free amino acid profles. The experimental fexibility ofered by our micro‑mash method allowed us to investigate the efects of altered mash parameters on the beer brewing proteome. Beer brewing is one of the oldest known biotechnologies and has heavily infuenced society and science. Te ancient science of beer brewing is rapidly developing, with modern analytical techniques allowing deeper under- standing of fundamental aspects of its integrated bioprocesses 1–10. Te brewing process begins with harvested bar- ley seeds being malted through a process which involves controlled, partial germination followed by kilning 11,12. Te malt is then milled to open the husks and combined with warm water in a process known as mashing. Mash- ing extracts the soluble sugars and proteins from the malt which are digested by enzymes into smaller sugars and free amino nitrogen (FAN)11. Wort, the soluble fraction of the mash, is then boiled with hops to introduce bitterness and aromatic favours, remove volatile of-favours, stop enzymatic activity, and sterilize11,13. Fermenta- tion is performed by pitching yeast into cooled wort, where the yeast consume the sugars and FAN and produce favour compounds, carbon dioxide, and ethanol. Finally, beer is fltered, aged, carbonated, and packaged. Complex and dynamic protein and carbohydrate chemistry occur during mashing and the boil1. Tese pro- cesses are very well understood from an industrial standpoint, but the underlying biochemical details are only starting to be appreciated11,12. Mashing extracts the soluble component from malted barley seeds, primarily starch and proteins, including enzymes 11. Extracted starch and proteins are then broken down by enzymes into small fermentable sugars and FAN, respectively11. Proteomics has been used to identify and monitor changes in the abundance of these enzymes and other proteins during the mash and boil and into the fnal beer product 1,4–10. Tese studies found that while some proteins were heat-stable and could survive being boiled, other proteins were only active and stable at low temperatures, while many proteins were covalently modifed by a variety of processes during brewing 1,4–10,14. Brewing processes at the scale of a large industrial brewery or even a smaller craf brewery are time consum- ing, ingredient intensive, and require specialised technical equipment not typically found in research laboratories. Tis large scale makes it difcult and expensive to perform appropriately designed experiments with sufcient statistical power to advise the brewing process. Our previous investigation of the beer brewing proteome identifed and measured the abundance of hundreds of proteins during the mash, and found dynamic changes in protein abundance due to solubilisation, proteoly- sis, and degradation1. Here, we re-interrogate these datasets from a 23 L mash and boil and a 1 mL benchtop micro-mash1. We investigate the two datasets in more detail to test the reliability and comparability of a 1 mL benchtop micro-mash to large scale brewing. We present a micro-scale mash protocol that can be performed using a benchtop shaker incubator, allowing for high-throughput mashing and easy sample replication for sta- tistical analysis (Fig. 1). School of Chemistry and Molecular Biosciences, The University of Queensland, St Lucia, QLD 4072, Australia. *email: [email protected] Scientifc Reports | (2021) 11:1480 | https://doi.org/10.1038/s41598-020-80442-7 1 Vol.:(0123456789) www.nature.com/scientificreports/ Figure 1. Process and throughput of micro-scale mash compared with a standard Braumeister mash. Results Protein abundance profles are similar in 23 L Braumeister and 1 mL benchtop mashes. We interrogated the abundance profle of each individual protein from a 23 L Braumeister mash and a 1 mL bench- top mash (Fig. 2A). Tese protein abundance profles showed that at both mash scales proteins increased in abundance as temperature increased and proteins were solubilised. As temperature continued to increase, the temperature-sensitive proteins reduced in abundance due to denaturation, aggregation, and precipitation (Fig. 2A). Some subtle diferences were apparent between the 23 L and 1 mL mash scales, with protein extrac- tion being slightly faster in the 1 mL micro-scale mash than in the 23 L Braumeister mash (Fig. 2A). However, the profle of a given protein was generally very similar at both 23 L and 1 mL mash scales, while the profles for diferent proteins were distinct. We next directly compared the performance of the two mash scales by calculating the diference in normalized abundance, subtracting the normalized abundance of each protein at each stage between the two mashes (Fig. 2B and Supplementary Table S1). Tis diference map was consistent with the overall protein abundance profles (Fig. 2A) and demonstrated that proteins were more efciently extracted in the 1 mL micro-scale mash, with increased levels at the end (35 min) of the 63 °C rest, although these diferences were not statistically signifcant (Fig. 2B). Tis diference map also demonstrated that the loss of protein at higher temperatures was less efcient in the 1 mL micro-scale mash than in the 23 L Braumeister mash (Fig. 2B). Tis diference was particularly apparent during the boil. Regardless of these minor diferences in the rates of extraction and denaturation, these side-by-side and diference comparisons of the two mash scales showed their protein-specifc abundance profles were very similar overall, with very few statistically signifcant diferences (Fig. 2). Proteolytically cleaved forms of peptides show similar profles in a 23 L Braumeister mash and a 1 mL benchtop mash. Proteolysis of barley seed storage proteins releases FAN for yeast to consume dur- ing fermentation and has also been shown to be a key driver of protein stability during mashing1,11. Barley seeds contain proteases which can proteolytically clip barley seed storage proteins. We previously found that in a 23 L Braumeister mash, the proteolytically clipped forms of proteins tend to be less stable at higher temperatures in the mash, which results in loss of these non-tryptic peptides and an increase in the relative abundance of the full-length tryptic peptides1. However, this efect is site-specifc, as some proteolytically clipped forms show high stability. We therefore compared this key feature of the dynamic mashing proteome at 23 L Braumeister and 1 mL micro mash scales. Tis analysis showed that the abundance of site-specifc proteolysis products was very similar between the mash scales, with only slight diferences detected at lower temperatures early in the mash (Fig. 3A,B). For proteolytic forms of the peptide family V122LVTPGQCNVLTVHNAPYCLGLDI145 from α-amylase/ trypsin inhibitor CMa (IAAA) we found almost identical peptide abundance profles between the two mash scales, with few statistically signifcant diferences (Fig. 3 and Supplementary Table S2). Te semi-tryptic forms V122LVTPGQCNVLTVH135 and V 122LVTPGQCNVL132 were slightly increased in abundance at lower tempera- tures in the 1 mL mash compared to the 23 L Braumeister mash, but these diferences converged at higher temper- atures later in the mash (Fig. 3A). We also found a high level of similarity in peptide abundance profles between the two mash scales for proteolytic forms from the peptide family K-C99NVNVPYTISPDIDCSR115-I from non- specifc lipid-transfer protein 1 (NLTP1) (Fig. 3B). Full tryptic peptide K-C99NVNVPYTISPDIDCSR115-I and the semi-tryptic form N 100VNVPYTISPDIDCSR115-I were slightly higher in abundance early in the 1 mL micro- mash, but again these diferences decreased as the mash progressed (Fig. 3B). At both mash scales, both of these peptide groups showed equivalent overall profles when comparing the dynamics of proteolysis and the resulting protein stability across the mash and boil (Fig. 3). Scientifc Reports | (2021) 11:1480 | https://doi.org/10.1038/s41598-020-80442-7 2 Vol:.(1234567890) www.nature.com/scientificreports/ Figure 2. Overall protein abundance profles are similar between a 23 L Braumeister mash and a 1 mL micro-scale mash. (A) Normalised abundance at each time point in 23 L Braumeister and 1 mL micro-scale mashes, for all proteins identifed. Relative protein abundance was calculated as protein intensity at each point relative to trypsin intensity at the same point. Normalised abundance was then calculated as the relative protein abundance at each point relative to the combined relative protein abundance across all points. (B) Diference of the normalised protein abundance means at each time point between 23 L Braumeister and 1 mL micro-scale mashes. Positive values (blue), higher in 23 L Braumeister; negative values (red), higher in 1 mL micro-scale. Bold highlights, statistically signifcant (p < 0.05). Scientifc Reports | (2021) 11:1480 | https://doi.org/10.1038/s41598-020-80442-7 3 Vol.:(0123456789) www.nature.com/scientificreports/ A L L L L L L L L L L L L L L L L L L L L L L 23 1m 23 1m 23 1m 23 1m 23 1m 23 1m 23 1m 23 1m 23 1m 23 1m 23 1m B L L L L L L L L L L L L L L L L L L L L L L 23 1m 23 1m 23 1m 23 1m 23 1m 23 1m 23 1m 23 1m 23 1m 23 1m 23 1m Figure 3.
Details
-
File Typepdf
-
Upload Time-
-
Content LanguagesEnglish
-
Upload UserAnonymous/Not logged-in
-
File Pages10 Page
-
File Size-