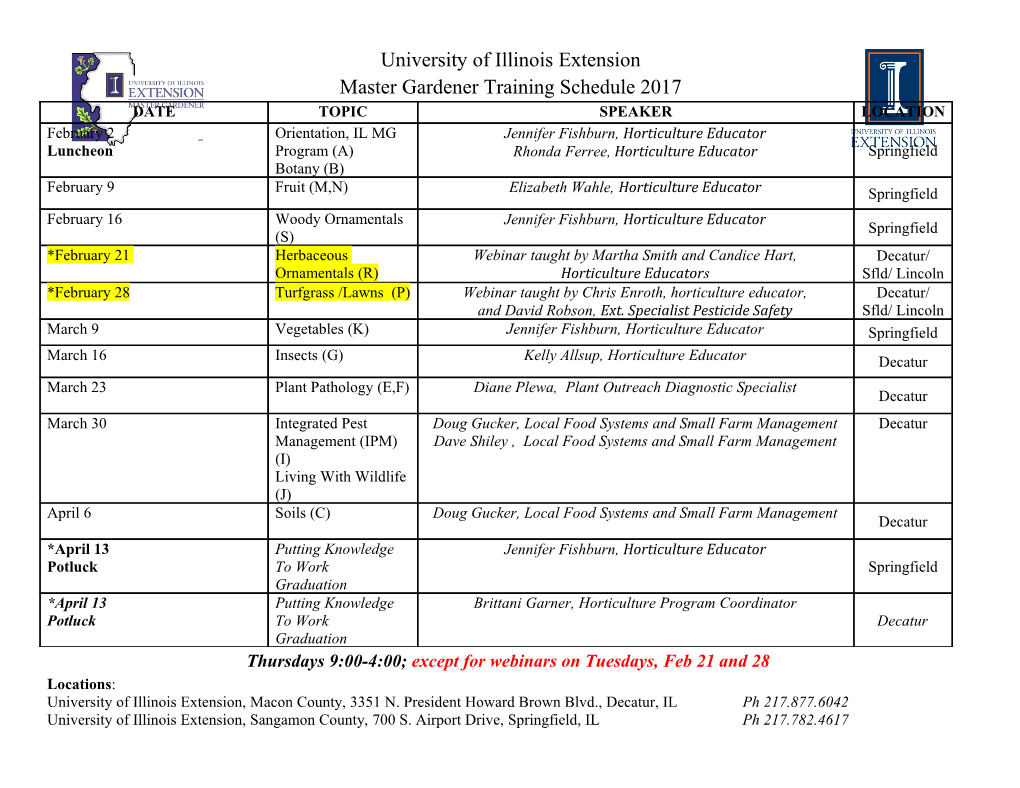
HHS Public Access Author manuscript Author ManuscriptAuthor Manuscript Author Nat Chem Manuscript Author Biol. Author Manuscript Author manuscript; available in PMC 2018 February 07. Published in final edited form as: Nat Chem Biol. 2017 October ; 13(10): 1088–1095. doi:10.1038/nchembio.2454. A genetically encoded tool for manipulation of NADP+/NADPH in living cells Valentin Cracan1,2,3,†, Denis V. Titov1,2,3,†, Hongying Shen1,2,3, Zenon Grabarek1,*, and Vamsi K. Mootha1,2,3,* 1Howard Hughes Medical Institute and Department of Molecular Biology, Massachusetts General Hospital, Boston, MA, USA 2Department of Systems Biology, Harvard Medical School, Boston, MA, USA 3Broad Institute, Cambridge, MA, USA Abstract NADH and NADPH are redox coenzymes broadly required for energy metabolism, biosynthesis and detoxification. Despite detailed knowledge of specific enzymes and pathways that utilize these coenzymes, a holistic understanding of the regulation and compartmentalization of NADH and NADPH-dependent pathways is lacking, in part because of a lack of tools with which to investigate them in living cells. We previously reported the use of the naturally occurring Lactobacillus brevis H2O-forming NADH oxidase (LbNOX) as a genetic tool for manipulation of the NAD+/NADH ratio in human cells. Here we present TPNOX (triphosphopyridine nucleotide oxidase), a rationally designed and engineered mutant of LbNOX that is strictly specific towards NADPH. We characterize the effects of TPNOX expression on cellular metabolism and use it in combination with LbNOX to show how the redox states of mitochondrial NADPH and NADH pools are connected. Graphical Abstract Users may view, print, copy, and download text and data-mine the content in such documents, for the purposes of academic research, subject always to the full Conditions of use: http://www.nature.com/authors/editorial_policies/license.html#terms *Corresponding authors: Vamsi K. Mootha, M.D., [email protected], Zenon Grabarek, Ph.D., [email protected], 185 Cambridge Street 6th Floor, Boston, MA 02114 USA. †These authors contributed equally to this work Author Contributions VC, DVT, ZG and VKM designed the study and interpreted data. VC and ZG performed the biochemical and structure-related experiments. DVT, VC, and HS performed cell-based experiments. VC, DVT, ZG and VKM wrote the manuscript. Data Availability Statement Protein coordinates and structure factors for LbNOX–NADH and TPNOX–NADPH have been submitted to the Protein Data Bank (PDB codes 5VN0 and 5VOH). Cracan et al. Page 2 Author ManuscriptAuthor Manuscript Author Manuscript Author Manuscript Author Introduction All forms of life require unremitting flux of energy, which is achieved through complex redox chemistry performed at the cellular level. Two pyridine dinucleotides, NADH and NADPH, are essential in this process due to their ability to serve as electron carriers for a large subset of oxidoreductases. Despite the identical redox chemistry of NAD+/NADH and NADP+/NADPH redox couples, and, consequently, similar standard reduction potentials (Eo′ = −320 mV), they work in their specific cellular environments at vastly different redox potentials (Eh), which are defined by the local concentrations of their oxidized and reduced forms. For example, in fed rat livers, the cytosolic free NAD+/NADH ratio is about 1000, + which corresponds to a reduction potential Eh = −240 mV, while the cytosolic free NADP / 1–4 NADPH ratio is ~0.01, which is equivalent to the reduction potential Eh ~ −400 mV . Cells maintain NADPH mostly in the reduced form to drive reductive biosynthesis and reactive oxygen species (ROS) detoxification, while the NADH pool is maintained mostly in the oxidized state to catalyze energy-producing reactions4. The preferential utilization of NADPH in anabolic processes and NADH in catabolic processes is an important design principle of cellular metabolism that allows thermodynamically incompatible reactions to take place simultaneously. The diverse metabolic functions of NADH and NADPH are possible due to the specificity and varied distribution of cellular enzymes that utilize these coenzymes. Though NADPH differs from NADH only by the presence of a single phosphate group in the 2′ position of the adenosine ribose, most human enzymes have evolved to selectively use either one or the other. Equally important is the compartmentalized distribution of these enzymes. For example, mammalian mitochondria, whose membrane is not permeable to pyridine nucleotides, maintain the NAD+/NADH pool in a more reduced state than in the cytosol. The difference is likely required to maintain thermodynamic favorability of oxidative phosphorylation in the mitochondria and glycolysis in the cytosol. The activity of core metabolic pathways and shuttle systems help to establish the observed NAD+/NADH and NADP+/NADPH reduction potentials in different compartments5. The NAD+/NADH ratio is determined by the relative activities of glycolysis in the cytosol, the tricarboxylic acid (TCA) cycle and electron transport chain (ETC) in mitochondria, as well as the activity of shuttle systems (e.g., aspartate–malate, glycerol-3-phosphate shuttles). The cytosolic NADP+/NADPH reduction potential is determined primarily by the pentose phosphate pathway (PPP), cytosolic malic enzyme, isocitrate dehydrogenase, and one- carbon metabolism pathway. In mitochondria, NADPH can be produced by transhydrogenase, malic enzyme, isocitrate dehydrogenase, and the one-carbon metabolism Nat Chem Biol. Author manuscript; available in PMC 2018 February 07. Cracan et al. Page 3 pathway. Furthermore, several shuttle systems for conversion of mitochondrial NADPH or Author ManuscriptAuthor Manuscript Author Manuscript Author Manuscript Author NADH into cytosolic NADPH have been proposed6,7. Knockout studies indicate redundancy in NADPH producing pathways5,8–10. It is not well-understood which of these pathways maintain the NADP+/NADPH reduction potential under different conditions. There is growing evidence that the regulation of NAD+/NADH and NADP+/NADPH reduction potentials is extremely important in physiology and disease. These ratios are highly dynamic, changing depending on the fasting/fed state1,2, diet1,2,11, exercise12, circadian rhythm13, cell proliferation status14 or pathologic states including cancer14, diabetes1 and the aging process itself15,16. There is also growing evidence that changes in pool sizes of pyridine nucleotides vary with disease processes17. To what extent these changes are reflective of underlying pathology versus drivers of pathology is not known. To address these questions, robust methods are needed for measuring and manipulating pyridine nucleotide ratios in living cells. In recent years there has been progress in the development of genetic reporters for detecting the dynamics of NAD+/NADH or NADP+/ NADPH in cells18–21. Mass spectrometry tracer methods for measuring fluxes through compartment specific NADH- and NADPH-producing pathways have also been developed22–25. However, methods for direct manipulation of NADH and NADPH levels in subcellular compartments are lacking. We recently reported the use of NADH oxidase from Lactobacillus brevis (LbNOX)26 which + + catalyzes a four-electron reduction of oxygen to water (2NADH + 2H + O2 → 2NAD + + 2H2O) as a genetically encoded tool for manipulation of NAD /NADH ratios in human cells. Here we report the design and validation of TPNOX, a quintuple mutant of LbNOX that is highly specific for NADPH. The X-ray structures of LbNOX–NADH and TPNOX– NADPH complexes provide new insights into the substrate specificity of H2O-forming oxidases. We show that TPNOX is active when expressed in mammalian cells and use it in combination with LbNOX to demonstrate that intra-mitochondrial but not intra-cytosolic NADPH and NADH reduction potentials are connected. Results Rational design of the water-forming NADPH oxidase TPNOX To our knowledge there are no naturally occurring H2O-forming oxidases that are highly + + specific for NADPH (2NADPH + 2H + O2 → 2NADP + 2H2O). Therefore, we sought to engineer an NADPH-specific mutant of the H2O-forming oxidase from L. brevis (LbNOX)26. Such an approach seemed feasible since several groups have had reasonable success in converting the NAD(P)H coenzyme specificities of various oxidoreductases27. The NADH binding site in LbNOX and related enzymes contains two defined motifs: a conserved pyridine dinucleotide binding motif (DBM) GxGxxG/A, which is a part of the βαβ Rossmann fold domain, and an adjacent motif designated as “substrate specificity loop” (SSL) (Fig. 1a)27. Comparison of the amino acid sequence of LbNOX with that of structurally related strictly NADPH-specific enzyme glutathione reductase (GR) and other related enzymes helped to identify five key residues spanning both motifs (numbered 1–5 in Nat Chem Biol. Author manuscript; available in PMC 2018 February 07. Cracan et al. Page 4 Fig. 1a). Based on the structure of LbNOX–NADH complex (determined in this study, see Author ManuscriptAuthor Manuscript Author Manuscript Author Manuscript Author below) and the structure of GR-NADPH (PDB code 1GET) we rationalized that positions 2, 3 and 5 contribute to the GR preference for the phosphate-bearing NADPH over NADH (Fig. 1b,c; Supplementary Results, Supplementary Fig. 3a and Supplementary Table 1). The negatively charged side chain of Asp177 (position 2) stabilizes NADH binding in LbNOX via two strong hydrogen bonds to the ribose hydroxyl groups in positions 2′
Details
-
File Typepdf
-
Upload Time-
-
Content LanguagesEnglish
-
Upload UserAnonymous/Not logged-in
-
File Pages25 Page
-
File Size-