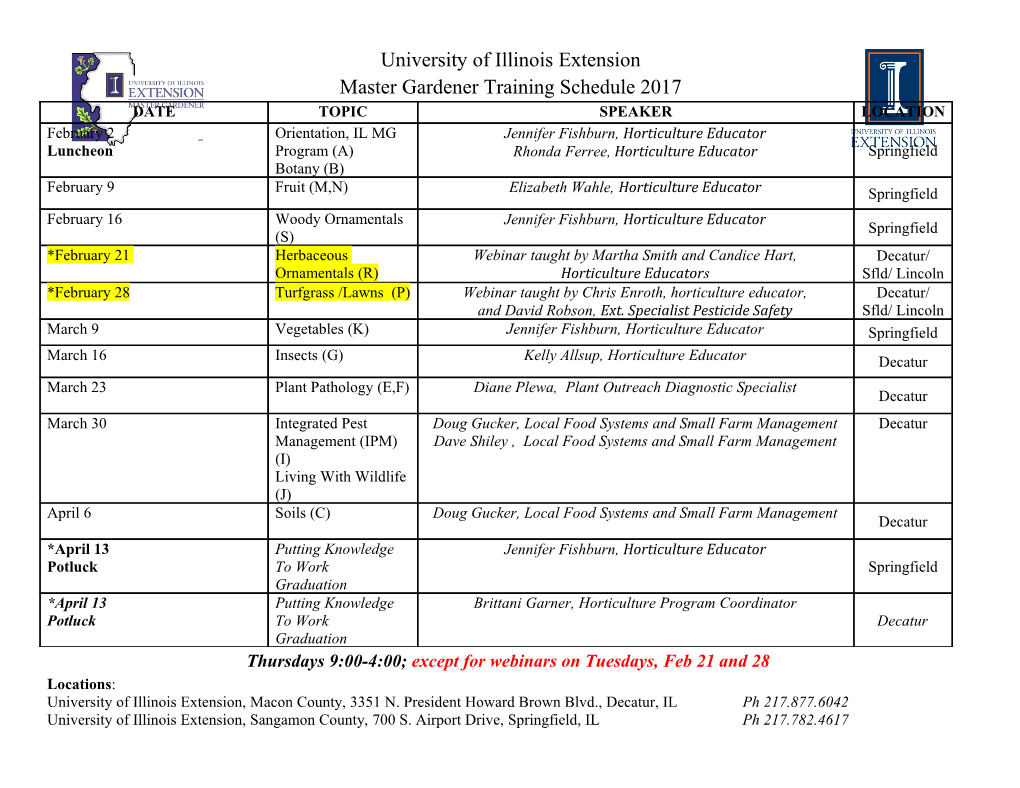
THE DETERMINATION OF THE FRACTURE ARCHITECTURE AND DEFORMATIONAL BEHAVIOR OF THE FISH LAKE VALLEY FAULT ZONE, CALIFORNIA: A DIGITAL APPROACH USING PHOTOREALISTIC SURFACE MAPPING WITH LIDAR by Rebecca Aguilar APPROVED BY SUPERVISORY COMMITTEE: ___________________________________________ Carlos L. V. Aiken, Chair ___________________________________________ John S. Oldow ___________________________________________ William R. Griffin Copyright 2017 Rebecca Aguilar All Rights Reserved Dedicated to my advisor Dr. Carlos Aiken, my lab mates Tiffany Savage, Tara Urbanski, Mansour Alhumimidi, my colleagues Bach Pham, Lionel White and my Mom, Lulu, Dad, Joel and sister, Amaya for their support and incredible advice. THE DETERMINATION OF THE FRACTURE ARCHITECTURE AND DEFORMATIONAL BEHAVIOR OF THE FISH LAKE VALLEY FAULT ZONE, CALIFORNIA: A DIGITAL APPROACH USING PHOTOREALISTIC SURFACE MAPPING WITH LIDAR by REBECCA AGUILAR, BS THESIS Presented to the Faculty of The University of Texas at Dallas in Partial Fulfillment of the Requirements for the Degree of MASTER OF SCIENCE IN GEOSCIENCES THE UNIVERSITY OF TEXAS AT DALLAS August 2017 ACKNOWLEDGMENTS I am eternally grateful for Dr. Carlos Aiken’s endless support and advice. I want to thank Bach Pham for being an excellent field partner and friend that helped me brave long days in the field. I also want to thank Tiffany Savage, Tara Urbanski, Mansour Alhumimidi, and Lionel White for their abundant intellect and advice. I want to thank Dr. John Oldow, the Miles Foundation, and Pioneer for providing resources, equipment and for funding this work. I would like to acknowledge R.W. Allmendinger for his Stereonet 9 software which was utilized in plotting fracture orientations. The concepts for the algorithms in Stereonet 9 can be found in Structural Geology Algorithms: Vectors & Tensors (Allmendinger, R. W. et al.) and Spherical projections with OSXStereonet (Cardozo, N., and Allmendinger, R. W., 2013). May 2017 v THE DETERMINATION OF THE FRACTURE ARCHITECTURE AND DEFORMATIONAL BEHAVIOR OF THE FISH LAKE VALLEY FAULT ZONE, CALIFORNIA: A DIGITAL APPROACH USING PHOTOREALISTIC SURFACE MAPPING WITH LIDAR Rebecca Aguilar, MS The University of Texas at Dallas, 2017 ABSTRACT Supervising Professor: Dr. Carlos L.V. Aiken Located in the Eastern California Shear zone (ECSZ), the Fish Lake Valley fault zone (FLVFZ) is a 250 km right-lateral fault and the longest active structure of the ECSZ. It offsets Pre- Cenozoic monzonite and metasediments and contains an estimated net displacement of 50 to 65 km. Just north of Death Valley, the FLVFZ passes through the Cucomungo Canyon Restraining Bend (CCRB), a step over 15 km wide. Recent uplift of the CCRB within the last million years has exposed Miocene and Pliocene deposits and internal structures of the fractures in the FLVFZ. The Mesozoic monzonites of the FLVFZ contain fault zones hundreds of meters wide consisting of alternating cataclasites and fractured rock. This work comprises a portion of the Miles Project, a multidisciplinary project implementing field mapping, Geophysics and terrestrial lidar scanning techniques to determine the deformation regime and motion of the faults. Mapping the well-exposed fractures in the CCRB with terrestrial lidar scanning serves as an example of the application of photorealistic surface models and tools to extract fracture structures and vi orientations in 3D. Building a photorealistic model consists of processing the lidar data, creating a triangulated irregular network mesh, georeferencing the site with global navigation satellite system coordinates and draping the photos onto the model. The mapped fracture patterns and orientations are utilized to determine the deformational behavior and movement in the fault zone. These data are combined with fieldwork to enable geologists to resolve and revisit field sites at any time. vii TABLE OF CONTENTS Acknowledgments…………………………………………………………………………….…..v Abstract…………………………………………………………………………………………...vi List of Figures…………………………………………………………………………………….ix Chapter 1: Introduction…………………………………………………………….……………...1 Chapter 2: Geology of the Fish Lake Valley Fault Zone………………………………………….4 Chapter 3: Photorealistic Surface Modeling from Lidar…………………………………………10 Chapter 4: GNSS Control………………………………………………………………………..19 Chapter 5 Mount Calibration and Vegetation Removal…………………………………………23 Chapter 6: Generating the TIN and the Photorealistic Model…………………………………...26 Chapter 7: Digitizing Fractures on Photorealistic Models……………………………………….31 Chapter 8: Results………………………………………………………………………………..35 Chapter 9: Conclusion and Discussion…………………………………………………………..45 References………………………………………………………………………………………..47 Biographical Sketch……………………………………………………………………………...51 Curriculum Vitae………………………………………………………………………………...52 viii LIST OF FIGURES Figure 2.1. A view of the western United States. Walker Lane and the ECSZ highlighted as active deformation zones (Oldow et al., 2008)…………………………………………………4 Figure 2.2. Hillshade map of the Fish Lake Valley fault zone with a draped DEM. FLV, Fish Lake Valley; FLVFZ, Fish Lake Valley fault zone; HTH, Horse Thief Hills; WW, Willow Wash; SM, Sylvania Mountains; CCRB, Cucomungo Canyon Restraining Bend; EV, Eureka Valley; LCR, Last Chance Range; DV-FCFS, Death Valley Furnace Creek fault system. Faults are outlined in black, blue arrows point to the dominant Fish Lake Valley fault strand………….5 Figure 2.3. View of the FLVFZ. Faults are outlined in red……………………………………….7 Figure 2.4. Geologic map of the FLVFZ. Pliocene sediments: tan, mid-late Miocene sediments: brown, Jurassic monzonite: blue, Paleozoic and Proterozoic metasediments: green……………..7 Figure 2.5. Close-up view of the study area with the scan sites. Faults outlined in red, blue arrows point to the dominant Fish Lake Valley fault strand………………………………………9 Figure 3.1. a. Close-up views of fractures on a photorealistic model. b. Comparison to a point cloud. Sedimentary beds: green, fractures: red, blue arrows point to the same feature, meter stick for scale…………………………………………………………………………………….12 Figure 3.2. a. Scan position of the Monzonite Fracture North outcrop viewed from the scanner’s position. b. Rotating the scan 90° to the right reveals occluded data (indicated by white arrows). c. Adding the data from a different scan position fills the holes from Figure 3.2.b……………..14 Figure 3.3. Looking east from above the Monzonite Fracture South point cloud with respective scan positions. White arrows point to holes in the data. Scan positions: grey rectangles, reflectors: red circles, black background: no data……………………………………………….16 Figure 3.4. Looking east on the Paleozoic Fracture point cloud. Scan positions: grey rectangles, reflectors: red circles. Black areas contain no data…………………………………..17 Figure 3.5. a. Looking east and from above the Monzonite Fracture North point cloud. b. Looking north into the canyon, Monzonite Fracture North is on the right side of the image. Scan positions: grey rectangles, reflectors: red circles, hill slope feature: highlighted in blue………..18 Figure 4.1. a. The GNSS positions of the base station and reflectors of the Paleozoic Fracture outcrop. b. The GNSS positions on Google Earth. The reflectors in Figures 4.1-4.3 correspond to the reflectors in Figures 3.3-3.5……………………………………………………………….20 ix Figure 4.2. a. The GNSS positions of the base station and reflectors of the Monzonite Fracture South outcrop. b. The GNSS positions on Google Earth. c. GNSS positions of the base station and the CORS P094 position. d. Comparison image on Google Earth…………………………..21 Figure 4.3. a. The GNSS positions of the base station and reflectors of the Monzonite Fracture North outcrop. b. The GNSS positions on Google Earth………………………………………...22 Figure 5.1.a. A side by side comparison of the reduction of pixel error before. b. After performing a mount calibration. Note how the “tp004” marker appears in the center of the reflector…………………………………………………………………………………………..24 Figure 5.2. The results of a mount calibration…………………………………………………...24 Figure 6.1. Close-up of the mesh exhibiting the surface artefact. Green circles: artefacts, red circles: “crumpled aluminum foil” features………………………………………………….26 Figure 6.2. a. Comparison of the TIN mesh before cleaning the mesh. b. The mesh after hole filling, and reducing the triangle count from 5.3 million to 1.3 million. White arrows point to the same feature……………………………………………………………………………….30 Figure 7.1. GAT’s Sedimentary Analysis Tools is utilized for outlining fractures and sedimentary beds. Red: Fractures, Blue-green: fault slip surface………………………………..31 Figure 7.2. a. From a distance, the fracture appears clearly defined (blue arrow) b. Up close, (blue arrow) part of the fracture is not discernable. Meter stick for scale………………………32 Figure 7.3. a. View with the model turned on. b. View with the model turned off. c. A profile view of the same fractures looking parallel to the fracture plane. View with the model turned on. d. View with the model turned off. Blue arrows point to the same feature, meter stick for scale………………………………………………………………………………………………33 Figure 7.4. GAT’s Structural Geology tools calculates the fracture’s orientation from the points placed on the fracture’s surface expression, assuming that fracture is a planar feature. It is not possible to obtain an orientation on nonplanar fractures or fractures that exhibited a change in direction……………………………………………………………………………….34 Figure 8.1. a. An overall view of the Paleozoic Fracture model. b. A close-up view of the fracture zone. Red: fractures, green:
Details
-
File Typepdf
-
Upload Time-
-
Content LanguagesEnglish
-
Upload UserAnonymous/Not logged-in
-
File Pages64 Page
-
File Size-