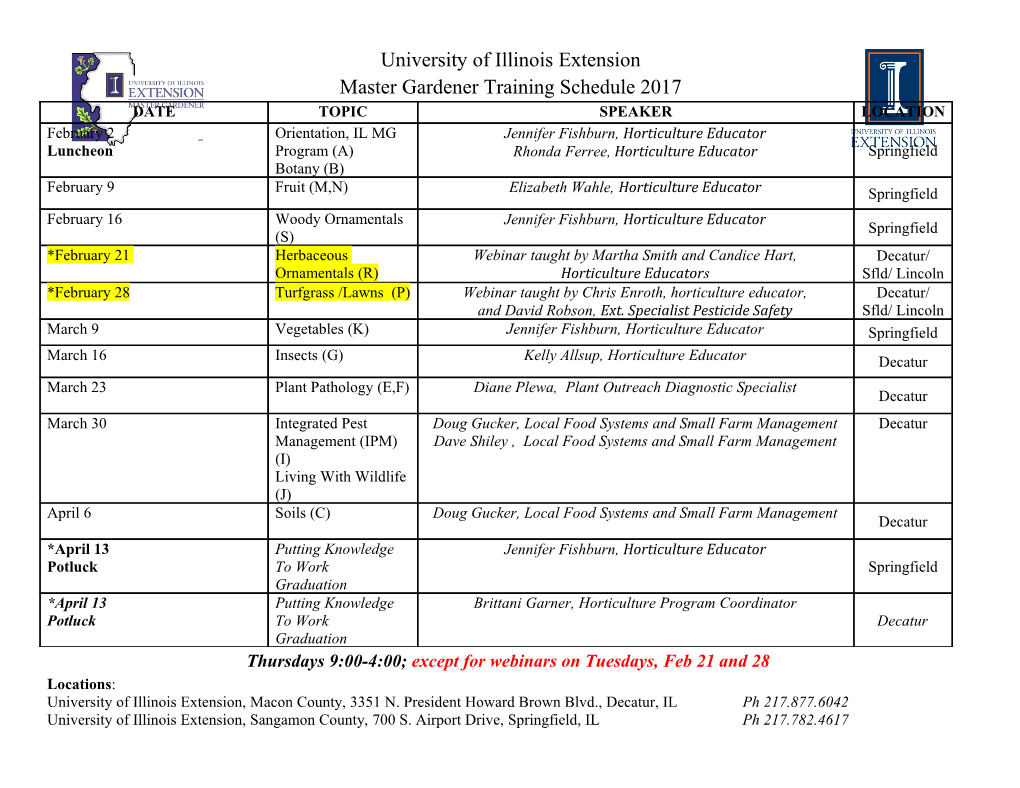
vol. 156, no. 4 the american naturalist october 2000 The Relative Importance of Competition and Predation Varies with Productivity in a Model Community Brendan J. M. Bohannan1,* and Richard E. Lenski2,² 1. Department of Biological Sciences, Stanford University, patterns in abundance, diversity, and community struc- Stanford, California 94305-5020; ture. Historically, ecologists have focused on single pro- 2. Center for Microbial Ecology, Michigan State University, East cesses such as competition or predation that may explain Lansing, Michigan 48824-1325 variation in these patterns (e.g., Brooks and Dodson 1965; Submitted May 3, 1999; Accepted May 19, 2000 Cody 1974). More recently, ecologists have recognized the importance of studying interactions between these pro- cesses and have begun to identify factors that may deter- mine the relative role each process plays (Leibold 1989; abstract: Recent theory predicts that productivity can in¯uence Power 1992). For example, recent theory predicts that pro- the relative importance of predation and competition in determining ductivity can in¯uence the relative importance of preda- patterns in abundance, diversity, and community structure. In low- tion and competition in determining community patterns productivity systems, competition is predicted to be the major in- (Holt et al. 1994; Leibold 1996). In low-productivity sys- ¯uence on community patterns, while at high productivity, the major tems, competition is predicted to be the major in¯uence in¯uence is predicted to be predation. We directly tested this theory using a laboratory model community. Our model community con- on community patterns, and organisms that are successful sisted of the bacteriophage T2 (a virus that feeds on Escherichia coli) at competing for resources are predicted to be dominant. and two populations of E. coli, in glucose-limited chemostats. One In contrast, in high-productivity systems, predation is pre- E. coli population consisted of individuals that were sensitive to pre- dicted to be the major in¯uence, and organisms that are dation by T2 (ªvulnerableº E. coli), and the other population con- successful at avoiding predation are predicted to be sisted of individuals that were partially resistant to predation by T2 dominant. (ªless vulnerableº E. coli). We manipulated productivity in this ex- periment by running replicate chemostats with different input con- These predictions come from theoretical studies of sim- centrations of glucose. Our observations were consistent with the- ple, abstract communities. For example, consider a simple oretical predictions. We observed the decline of the more vulnerable community that consists of two prey species that share a prey population at higher productivity but not at lower productivity, common resource and predator (®g. 1). Let us assume that and the decline of the less vulnerable prey population at lower pro- there is a trade-off between being successful at competing ductivity but not at higher productivity. However, the rate of decline in some replicates was slower than predicted, and extinctions were for resources (ªexploitation abilityº) and being successful not observed during the experiments, contrary to theoretical pre- at avoiding predation (ªpredator resistanceº), such that dictions. We present some testable hypotheses that might explain the the better exploiter is least resistant to predation. There is slow rate of decline observed. evidence that such trade-offs exist (Lenski 1988; Simms 1992; Grover 1995; Kraaijeveld and Godfray 1997). At low Keywords: bacteria, bacteriophage, productivity, competition, pre- levels of productivity (e.g., region I in ®g. 2A), theory dation, community structure. predicts that the better exploiter (species A) will exclude the more resistant prey species (species B), through a com- bination of resource and ªapparentº competition (sensu One of the great challenges facing ecologists is to link Holt 1977). At high levels of productivity (e.g., region III pattern to process. This is especially dif®cult in community in ®g. 2A), theory predicts that the more resistant prey ecology, where multiple processes can interact to produce (species B) will exclude the better exploiter (species A), * E-mail: [email protected]. again through a combination of resource and apparent ² E-mail: [email protected]. competition. At intermediate levels of productivity (e.g., Am. Nat. 2000. Vol. 156, pp. 329±340. q 2000 by The University of Chicago. region II in ®g. 2A), theory predicts that both prey species 0003±0147/2000/15604-0001$03.00. All rights reserved. may coexist due to the trade-off between exploitation abil- 330 The American Naturalist Figure 1: Simple community in which two prey types (NA, NB) share a common resource (R) and a common predator (P). A trade-off between competitive ability for the resource and resistance to predation is as- sumed. The thick lines linking NA to R and P indicate that NA is a better competitor for resources and more susceptible to predation. Conversely, the thin lines linking NB to R and P indicate that NB is an inferior competitor for resources and less susceptible to predation. This simple community assumes that interference competition does not exist between prey types or among predators and that higher-order predators (i.e., consumers of P) do not exist. ity and predator resistance. The conditions for equilibrium coexistence are shown graphically in ®gure 2B. The general patterns predicted by this theory are con- sistent with observations of some natural communities. For example, this theory predicts the following three gen- eral patterns (®g. 2A). First, the biomass of total prey and predator will both increase, in a steplike manner, in re- sponse to increased productivity. Second, the relative bio- mass of predator-resistant species will increase as produc- tivity is increased. Third, the diversity of prey species will ®rst increase then decrease as productivity is increased, resulting in a hump-shaped relationship between diversity and productivity. These patterns are predicted even if the models are expanded to include multiple prey types and spatial patchiness (Leibold 1996). These general patterns have been observed in some natural communities, partic- Figure 2: Effect of productivity on the density of two prey types, a shared ularly freshwater lentic communities. Positive correlations predator, and a shared resource. Productivity is assumed to be propor- between biomass at adjacent trophic levels in response to tional to resource input concentration. A, Predicted pattern of equilib- rium population densities across a gradient of productivity (modi®ed changes in productivity have often been observed (Leibold from Leibold 1996); RP¤¤p equilibrium resource concentration; p 1989, 1996). The biomass of predator-resistant species has ¤ p equilibrium predator density; NA equilibrium density of more vul- ¤ p also been observed to increase as productivity increases nerable prey; NB equilibrium density of less vulnerable prey. B, Graph- (Watson et al. 1992). Hump-shaped relationships between ical analysis of an idealized community model (modi®ed from Leibold diversity and productivity have been observed in a number 1996) showing zero net growth isoclines (ZNGI) and net consumer-prey impact vectors (C) for two prey types (A and B). Coexistence is possible of studies (reviewed in Rosenzweig 1995). because the ZNGIs intersect. The zone of coexistence is determined by However, this theory has not been tested directly. In this the slope of the impact vectors and their intersection with the resource article, we report a direct test of this theory using a labo- axis. Productivity, Predation, and Competition 331 ratory model community. We assembled communities that chains (Bohannan and Lenski 1997) and in communities consisted of bacteriophage T2 and two Escherichia coli with vulnerable and invulnerable prey (Bohannan and strains that differed in their susceptibility to this bacterio- Lenski 1999). phage. We manipulated the productivity of this model com- munity and compared the observed responses to the pre- dictions of a mathematical model that includes both Methods competition for resources and avoidance of a shared Model Community predator. Chemostat communities of E. coli and bacteriophage T2 Our model community consisted of Escherichia coli B are excellent model systems with which to test shared pred- strain REL607 (Lenski et al. 1991), E. coli B strain REL6584 ator/shared resource models for a number of reasons. First, (Bohannan and Lenski 1999), and the virulent bacterio- T2 is one of the few bacteriophages against which E. coli phage T2 (provided by L. Snyder) in glucose-limited che- has been observed to evolve partial resistance (i.e., reduced mostats. Strain REL6584 is identical to REL607 with the vulnerability; Lenski 1984). Escherichia coli can evolve exceptions that it cannot utilize the sugar arabinose and complete resistance (i.e., invulnerability) to many bacte- it is invulnerable to predation by bacteriophage T4. We riophages; however, partial resistance is rarely observed. used the ability to utilize arabinose as a neutral marker to Because partial resistance against T2 is possible, model distinguish the two E. coli strains. We previously checked communities that consist of prey populations that differ the neutrality of the arabinose-utilization marker, and we quantitatively in susceptibility to a shared predator can be detected no effect of the arabinose-utilization marker on constructed
Details
-
File Typepdf
-
Upload Time-
-
Content LanguagesEnglish
-
Upload UserAnonymous/Not logged-in
-
File Pages12 Page
-
File Size-