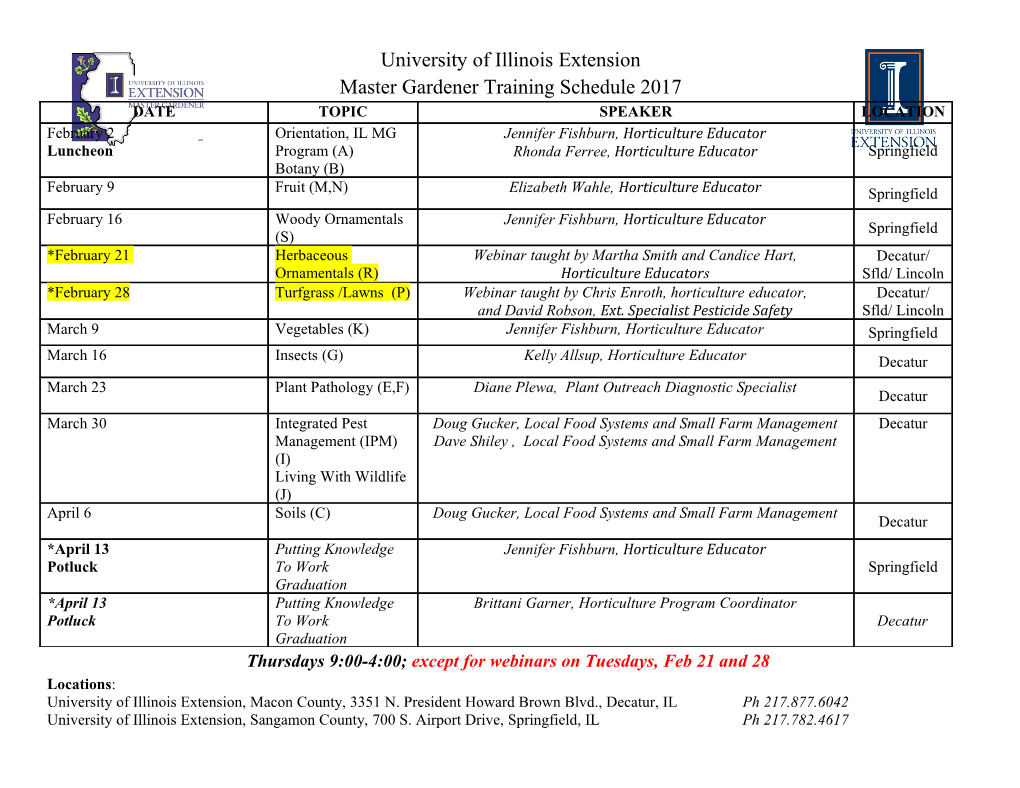
MAY 2004 KLYMAK AND GREGG 1135 Tidally Generated Turbulence over the Knight Inlet Sill JODY M. KLYMAK College of Oceanic and Atmospheric Sciences, Oregon State University, Corvallis, Oregon MICHAEL C. GREGG Applied Physics Laboratory and School of Oceanography, University of Washington, Seattle, Washington (Manuscript received 14 June 2002, in ®nal form 18 November 2003) ABSTRACT Very high turbulent dissipation rates (above «51024 Wkg21) were observed in the nonlinear internal lee waves that form each tide over a sill in Knight Inlet, British Columbia. This turbulence was due to both shear instabilities and the jumplike adjustment of the wave to background ¯ow conditions. Away from the sill, turbulent dissipation was signi®cantly lower («51027 to «51028 Wkg21). Energy removed from the barotropic tide was estimated using a pair of tide gauges; a peak of 20 MW occurred during spring tide. Approximately two- thirds of the barotropic energy loss radiated away as internal waves, while the remaining one-third was lost to processes near the sill. Observed dissipation in the water column does not account for the near-sill losses, but energy lost to vortex shedding and near-bottom turbulence, though not measured, could be large enough to close the energy budget. 1. Introduction straight section containing a large sill. They hypothe- Tides are an important source of energy in the oceans, sized that the highly turbulent nonlinear lee waves near both in the major basins and in marginal seas and es- the sill (Farmer and Smith 1980; Farmer and Armi tuaries. Tidal energy is dissipated directly by bottom 1999a; Klymak and Gregg 2003) were responsible for friction or via form drag due to internal waves or vortical dissipating most of the energy. Subsequent work by Sta- motions. Tidally generated internal waves are a possible cey (1985) argued that the energy withdrawn from the source of energy that drives mixing in the deep abyssal tide can be accounted for using a linear internal wave ocean (Munk and Wunsch 1998). Vortical motions, cre- model (Stigebrandt 1980). This model has not been test- ated by ¯ow separations around headlands, have been ed with observational data and the application of a linear observed in coastal waters (Signell and Geyer 1991; internal wave model is suspect. Klymak and Gregg 2001), but their importance to en- This paper presents the ®rst systematic measurements ergy budgets rarely considered. of turbulence in Knight Inlet, and one of the few ex- Energy balances are dif®cult to calculate in the open amples of turbulence measurements in a fjord. Gargett ocean, whereas the con®nes of a coastal fjord are ideally (1985) presented data collected in Knight Inlet from a suited to such studies. The barotropic tide in a deep submarine, but that experiment was designed to test high fjord will typically behave like a nearly perfect standing Reynolds number turbulence rather than to constrain the wave; much of the barotropic tidal energy entering the energy near the sill. Here over 745 pro®les were used fjord is re¯ected out again. The re¯ection, however, is to measure the dissipation of turbulent kinetic energy never 100% ef®cient, and some energy is lost to bottom near the sill, with particular focus on the energetic non- friction or internal motions. The energy budget of linear lee waves. The direct turbulent dissipation data Knight Inlet, a fjord in central British Columbia, Can- was supplemented by another 3400 CTD casts from ada, has been studied extensively. Freeland and Farmer which the dissipation rate was estimated using the over- (1980) calculated the energy lost from the barotropic turning method of Thorpe (1977). tide between two pairs of tide gauges in the inlet and After a brief description of Knight Inlet and the meth- found that the majority of the energy was lost in a ods used to measure the ¯ow and turbulence near the sill (section 2), we present detailed dissipation mea- surements (section 3). The detail is necessary because Corresponding author address: J. Klymak, Scripps Institution of Oceanography, UCSD, 9500 Gilman Dr., Mail Code 0213, La Jolla, the strong temporal and spatial dependence of nonlinear CA 92093-0213. ¯ows do not average well, and some features were cap- E-mail: [email protected] tured in very few events. Furthermore, we wish to justify q 2004 American Meteorological Society Unauthenticated | Downloaded 10/02/21 09:13 AM UTC 1136 JOURNAL OF PHYSICAL OCEANOGRAPHY VOLUME 34 FIG. 1. Knight Inlet, BC, Canada, a sinuous fjord 300 km north of Vancouver. our use of the overturning method to estimate dissipa- tion, increasing our statistics. An energy budget of the inlet is constructed by comparing the energy lost from FIG. 2. (top) Topography of the Knight Inlet sill. Contour intervals the barotropic tide with energy radiated in internal are 50 m. The locations of instrument deployments for the cruise are shown as shaded dots. The sill crest is at 0 km west±east and is waves and energy lost from the ¯ow near the sill (section bracketed by headlands to the north and south. Seaward is to the 4). A conclusion and discussion of the energy balance west. (bottom) Sill depth along the deepest portion of the inlet. Con- are offered in section 5. tours are of density from early ¯ood tide. 2. Site and data collection and standard microstructure measurements [see Wesson Knight Inlet is a fjord 300 km north of Vancouver, and Gregg (1994) for details about this instrument]. British Columbia, stretching 100 km into the mainland Near the sill, SWIMS, AMP,and Vector CTD casts were from the Queen Charlotte Strait (Fig. 1). The inlet is 2± made east±west along the inlet axis, except for one day 3 km wide with vertical sidewalls. A sharp sill 25 km during ¯ood tide when the R/V Miller ran a series of from the mouth divides the inlet into a seaward basin cross-inlet lines (Fig. 2). Note that the deepest part of 160 m deep and a landward basin 450 m deep. A river the inlet on the landward side of the sill is actually at the head of the inlet provides a strong buoyancy slightly south of the along-inlet lines. Both the R/V source during summer when it is fed by glacier melt. Miller and C.S.S. Vector were equipped with Biosonics The semidiurnal tide drives mean currents approaching 120-kHz echo sounders, capable of resolving backscat- 0.7ms21 over the sill, generating strong nonlinear in- ter from small biology and microstructure in the water ternal lee waves. These waves were the focus of a recent column (Seim et al. 1995). Farmer and Armi (1999a) observational program that examined the time depen- present a number of these echo-sounder images from dence (Farmer and Armi 1999a; Klymak and Gregg the C.S.S. Vector. 2003) and the three-dimensionality of the ¯ow (Klymak We also deployed two SeaBird tide gauges for the and Gregg 2001). duration of the cruise, one 9.8 km west of the sill and The data presented here were collected 18 August±3 the other 2.5 km east. These gauges measured the pres- September 1995 from the C.S.S. Vector, equipped with sure at hourly intervals using a 60-s-long burst sampled a 300-kHz RD Instruments (RDI) Broadband (BB) at 1 Hz and then averaged to give an accuracy of 0.5 ADCP to measure the currents, a Guildline CTD to mea- cm. Tidal heights were estimated by removing the sure density, and a GPS for position determination, and cruise-mean pressure signal; a harmonic analysis (dis- from the R/V Miller equipped with a GPS and a 150- cussed below) shows a phase lag between the two gaug- kHz RDI BB ADCP. ADCP performance on the Miller es due to energy withdrawn from the barotropic tide. was suboptimal for most of the cruise; noise was not The primary method for estimating turbulence was averaged out satisfactorily. Two pro®ling packages were our loosely tethered dissipation pro®ler AMP. The AMP deployed from the Miller. The ®rst, the Shallow Water and how it is used to calculate turbulent dissipation are Integrated Mapping System (SWIMS), is a towed body described in Moum et al. (1995) and Wesson and Gregg equipped with two SeaBird 911 CTDs designed to point (1994). Brie¯y, it measures small-scale velocity ¯uc- into clear water while being towed up and down di- tuations as it falls through the water by measuring the agonally through the water as the ship steamed at 1 m change of the ¯ow direction on a small airfoil. A spec- s21. The second, the Advanced Microstructure Pro®ler trum of turbulent shear can be estimated if the velocity (AMP), is a loosely tethered pro®ler capable of CTD of water past the probe (w), the probe's sensitivity, and Unauthenticated | Downloaded 10/02/21 09:13 AM UTC MAY 2004 KLYMAK AND GREGG 1137 its response to velocity ¯uctuations are known. The shear spectrum is then integrated to give the turbulent dissipation rate «, presented here in units of watts per kilogram. The only measurement dif®culty was esti- mating the speed of the ¯ow past the pro®ler. This is usually done by differentiating the pressure signal, but this at times required correction to account for the very strong vertical velocities observed in Knight Inlet. The turbulent dissipation rate depends inversely on the mag- nitude of this velocity to the fourth power (« ø w 24), and this correction is signi®cant for a number of drops in the lee wave (see the appendix). Few AMP casts were made, and their temporal res- olution was poor in comparison with tow-yoed CTDs.
Details
-
File Typepdf
-
Upload Time-
-
Content LanguagesEnglish
-
Upload UserAnonymous/Not logged-in
-
File Pages17 Page
-
File Size-