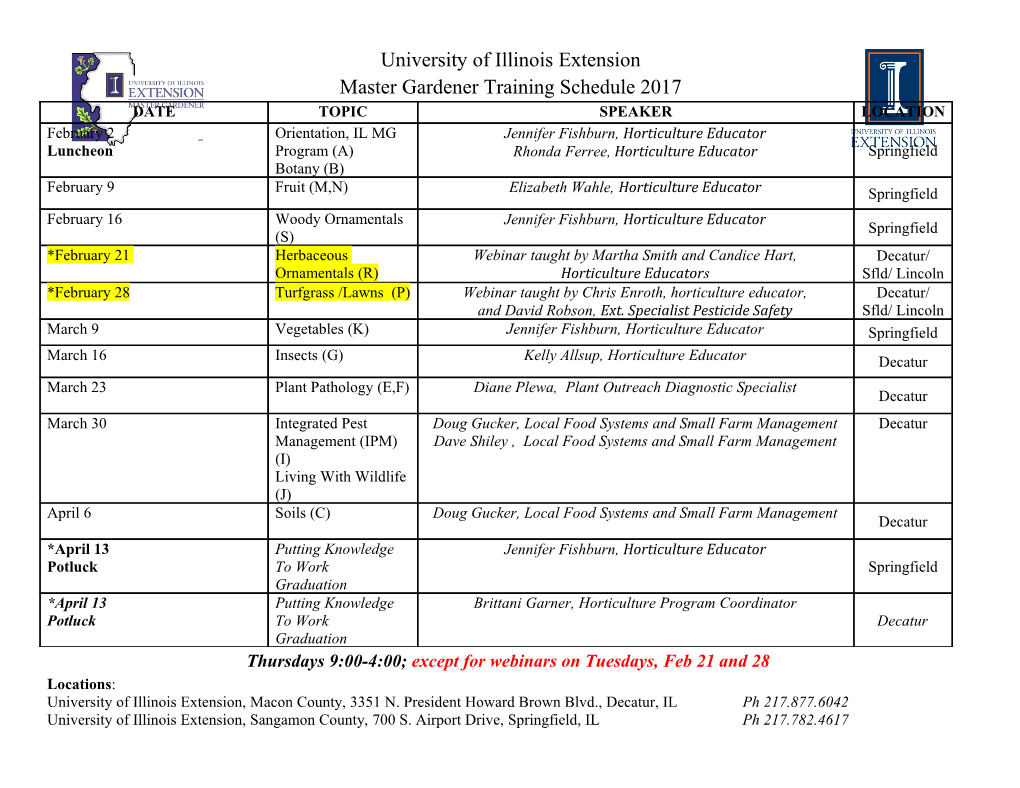
Headline THE DISCOVERY of technetium-99m and iodine-131. Authors Sergio Modoni (1) Nicoletta Urban (2) Luigi Mansi (3) (1) Nuclear Medicine, Oncological Referral Center of Basilicata, Rionero in Vulture (PZ) (2) Division of Nuclear Medicine, European Institute of Oncology, Milan. (3) Chair of Nuclear Medicine, Faculty of Medicine and Surgery, 2nd University of Naples. For correspondence: Sergio Modoni Unit of Nuclear Medicine Oncological Referral Center of Basilicata Prov.le Road 8 85028 Rionero in Vulture (PZ) tel.: 0972 726340 Mobile. 333 2822062 e mail: [email protected] Introduction Technetium-99m (99m Tc) and iodine-131 (131 I) are the everyday tools of Nuclear Physician. The discovery of these radioisotopes, as well as constitute a crucial step in the history of radiation in medicine, it also intersects with crucial events of the last century, with the most important discoveries in the field of physics of radiation, which is no stranger to the Italian genius, and events that have improved and sometimes shocked, but determined the history of humanity. Some premises of physics In the light of the knowledge acquired, we now know that the nucleus of an atom there are Z protons and N neutrons and Z that identifies the atomic number and the mass number A is given by the sum of N + Z, ie the number of protons and neutrons present in the nucleus of that element. Therefore, a nuclide of an element is identified by the values of A and Z and its symbol is: Nuclides with the same atomic number (Z) and different mass number (A) are called isotopes: Until 1919, the only known nuclear phenomena were those related to natural radioactivity produced by the last 12 elements of the periodic system, with Z from 81 to 92. Today there are more than 1400 nuclides. Each radioactive isotope is characterized by certain physical parameters which enable the identification: the physical half-life, ie, the time in which the original radioactivity is halved, the type of radioactive emission (alpha, beta, gamma) and the energy of the the emitted radiation. In the case of radioisotopes which we speak of these characteristics are shown in Table 1. The Birth of Nuclear Medicine Although the use, for therapeutic purposes, of compounds containing radio goes back to the early 1900's, we can align the birth of nuclear medicine with the discoveries of Georg de Hevesy, Hungarian physicist, of noble family, who went to work with Rutherford at 'University of Manchester. At the time, Rutherford was studying the radioactive properties of what was then known as the Radium-D (today would be the Lead-210). To complicate his life and his studies, lead present in Radium-D interfered with his analysis. Not yet aware that the Radium-D was an isotope of lead, Rutherford thought he could isolate and chemically, therefore, entrusted this task to de Hevesy, saying: "My boy, if you are worth your salt, you try to separate radium -D from all that lead ". [1] Ironically, this was his inability to lead out the task entrusted to him, which allowed one of the greatest discoveries in the field of radioactive tracers, for which Georg de Hevesy today is considered the Father of Nuclear Medicine. In fact, he thought of using radioisotopes to study the biological behavior of their stable isotopes. Therefore performed studies on plants and animals and finally employed the water containing deuterium (an isotope of hydrogen) to study the turnover of the water in the human body. In 1935, together with O. Chieivitz administered phosphate labeled with phosphorus-32 to rats and demonstrated the renewal of the mineral components of bone and in this way laid the groundwork for what would become the radionuclide therapy of bone metastases, widely used today. With his studies, de Hevesy paved the way for the use of radioisotopes as "tracers" of metabolic pathways in the human body. For these studies, in 1943 he was awarded the Nobel Prize for chemistry. The first "particle accelerators" In the 20s, the only method available for the study of the core was developed by Rutherford, who was to bombard the nuclei with alpha particles. But the repulsive forces between the nuclei and alpha particles, both positively charged, and the low energies of the latter, did not allow good results especially with elements of high atomic number. In 1929 Ernest Orlando Lawrence, UC Berkeley, he began to develop the idea of the cyclotron noting that potassium ions that passed through two metal tubes subjected to oscillating voltage were accelerated and emerged with an energy twice that of entry. Lawrence built two rooms in the form of D (from which the name of "dees"), and placed between the poles of a magnet. Inside the "dees" ions were accelerated on a spiral path and then extracted with a very high energy. Between 1931 and 1940 Lawrence cyclotrons built bigger and bigger. Suffice it to say that the first cyclotron 80,000 eV staying in the palm of a hand, while the last, from 100 million electron volts, could accommodate dozens of people inside the magnet. Lawrence took these cyclotrons to study nuclear processes and to produce a variety of new isotopes, some of which are very important for medicine, so much so that in 1935 he had this to say: "Shall we call it nuclear physics or shall we call it nuclear chemistry ? ". For this work, Lawrence received in 1939 the Nobel Prize for Physics. The discovery of artificial radioactivity Another important finding, after the natural radioactivity, has marked the development of knowledge of modern physics. E 'on the afternoon of December 31, 1933. Irene Curie, daughter of Marie, who has followed in the footsteps of his mother, and her husband Frederick Joliot, are coming out of their lab to go home to celebrate the New Year, when a hastily called by their assistant who noted the presence of radioactivity of uncertain origin. Let's see how they themselves describe the discovery of artificial radioactivity: "... when an aluminum foil is irradiated by a preparation of polonium, the emission of positrons does not cease immediately with the removal of the active preparation. The foil remains radioactive and the emission decays exponentially ... We have proposed for the new radioactive elements ... the name radioazoto, radiosilicone, and radiophosphorus. These and other elements can be formed by bombarding with other particles: protons, deuterons, neutrons ... "[2]. And the neutrons are the basis of a Italian story, which became known to the world the value of our physics. And 'the story of Enrico Fermi and the Group of Panisperna that, under the enlightened guidance of Orso Mario Corbino, including Rasetti, Emilio Segre, Ettore Maiorana, Edoardo Amaldi, Bruno Pontecorvo and Gian Carlo Wick. It 's the story of the discovery, but intuitive reasoned, of slow neutrons, which led to a step from the discovery of nuclear fission then demonstrated by Lise Meitner, Otto Hahn and Fritz Strassmann. From this story comes the Appendix important for nuclear medicine, which can be placed as a source in 1925, and sees Emilio Segre, one of the main collaborators of Enrico Fermi at the center of these events. The discovery of technetium Before 1925, all stable elements found in nature had been discovered. The elements with atomic numbers 43, 61, 85 and 87 were "missing" because they were only radioactive. In that year, two German chemists, Ida Tacke and Walter Noddack, reported the discovery in some minerals, element 43, which they called masurium and, two years later, the element 75, which they called Rhenium, in honor of the eastern borders (the Masuri lakes) and western (the Rhine) in Germany. These names were not without a certain nationalistic spirit since in these regions the German troops during the First World War, had achieved important victories. The two discoverers, however, they made no mention that the element 43 was radioactive. While the discovery of rhenium was confirmed and were prepared significant amounts, the masurium was ignored for several years and the spouses themselves Noddack-Tacke, especially because they were not able to document their discovery, they were ignored by the mainstream physics even when they provided, probably for the first, the correct scientific explanation of nuclear fission in 1938. So when, in 1936, Emilio Segre in Rome was working on the element 43, as he himself points out, he found the masurium: "I often had the task of procuring the necessary things to work. Luckily there was no bureaucracy. For chemicals ... I turned to Mr. Troccoli, an old shopkeeper highly competent in this field, he was proud to have a rich collection of rare substances also. He had studied at the seminary and liked to speak Latin, offering every so often, gratis et amore gods, some product that he had kept on his shelves for years without smerciarlo. The good man helped me in every possible way, especially after he had explained what we were doing. Only when in my ignorance I asked a sample of masurium, told me that he had never seen that element, "numquam I saw." A few years later I had to know what was right: masurium does not exist. "[3]. Although skeptical of this discovery, however Segre wanted to avoid a confrontation, partly because of the political situation in Europe. He tells the story in his autobiography so American, "was in 1938. There were two German chemists who claimed their 'discovery'. Who was I, an Italian physicist in Sicily [in the period 1936-38 Segre directed the Institute of Physics in Palermo, ed] to contradict them? I would be a fool to do it! I did not want to enter into the dispute related to this discovery, that time would prove to be erroneous.
Details
-
File Typepdf
-
Upload Time-
-
Content LanguagesEnglish
-
Upload UserAnonymous/Not logged-in
-
File Pages13 Page
-
File Size-