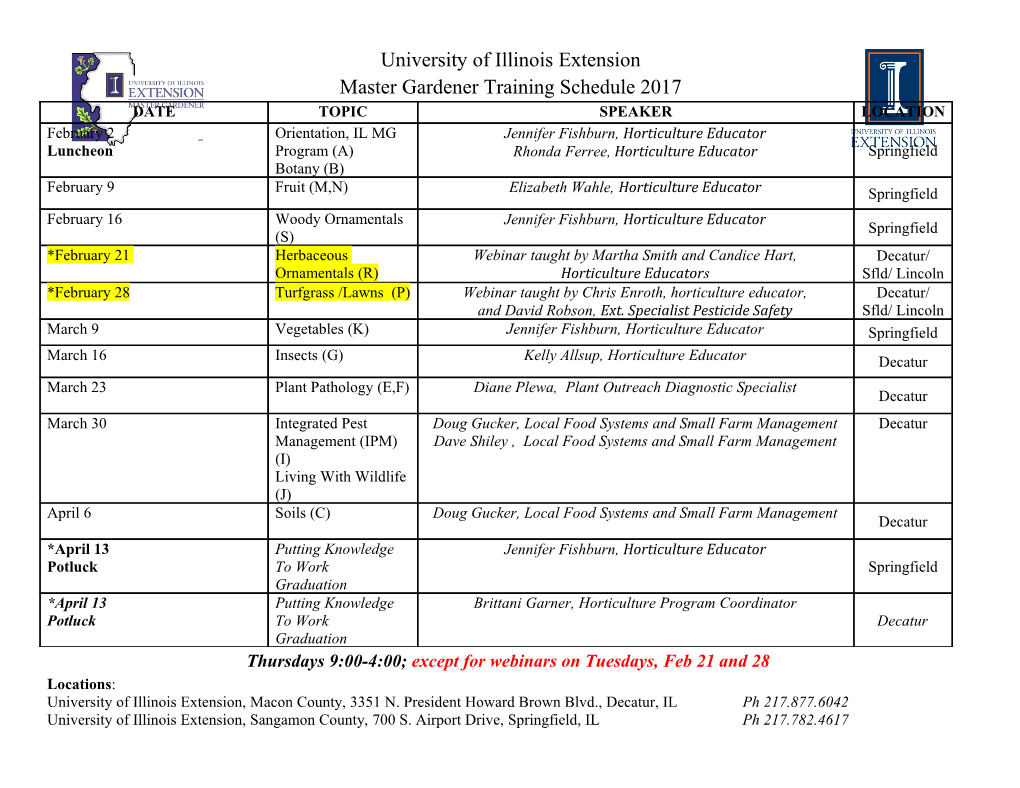
Downloaded from http://cshperspectives.cshlp.org/ on September 25, 2021 - Published by Cold Spring Harbor Laboratory Press Translation Elongation and Recoding in Eukaryotes Thomas E. Dever,1 Jonathan D. Dinman,2 and Rachel Green3 1Eunice Kennedy Shriver National Institute of Child Health and Human Development, National Institutes of Health, Bethesda, Maryland 20892 2Department of Cell Biology and Molecular Genetics, University of Maryland, College Park, Maryland 20742 3Department of Molecular Biology and Genetics, Johns Hopkins University School of Medicine, Baltimore, Maryland 21205 Correspondence: [email protected]; [email protected]; [email protected] In this review, we highlight the current understanding of translation elongation and recoding in eukaryotes. In addition to providing an overview of the process, recent advances in our understanding of the role of the factor eIF5A in both translation elongation and termination are discussed. We also highlight mechanisms of translation recoding with a focus on ribo- somal frameshifting during elongation. We see that the balance between the basic steps in elongation and the less common recoding events is determined by the kinetics of the different processes as well as by specific sequence determinants. OVERVIEW OF TRANSLATION cognate elongating aminoacyl-tRNA (transfer ELONGATION RNA) to the A site of the ribosome (Fig. 1). The eukaryotic translation elongation factor he mechanism of translation elongation is eEF1A, like its bacterial ortholog EF-Tu, is acti- Tconserved in all kingdoms of life. Whereas vated upon binding guanosine triphosphate most of the mechanistic details of the process (GTP) and forms a ternary complex upon bind- have been elucidated in studies of bacterial ing an aminoacyl-tRNA. The eEF1A•GTP•ami- translation (see Rodnina 2018), the key steps noacyl-tRNA complex binds in the A site. Base- are shared between eukaryotes and bacteria. In pairing interactions between the anticodon of eukaryotes, translation initiation culminates the aminoacyl-tRNA and the A-site codon trig- with formation of an 80S initiation complex in ger GTP hydrolysis by eEF1A. The eEF1A•GDP Met which Met-tRNAi is bound in the P (pep- complex is released and the aminoacyl-tRNA is tidyl) site of the ribosome. The anticodon of accommodated into the A site. Met the Met-tRNAi is base-paired with the start High-resolution cryo-electron microscopy codon of the messenger RNA (mRNA), and the (EM) structures of decoding complexes have second codon of the open reading frame (ORF) provided insights into how the bacterial and eu- is in the A (aminoacyl) site of the ribosome. karyotic ribosomes sense proper decoding (Jobe Elongation commences with delivery of the et al. 2018). The 18S ribosomal RNA (rRNA) Editors: Michael B. Mathews, Nahum Sonenberg, and John W.B. Hershey Additional Perspectives on Translation Mechanisms and Control available at www.cshperspectives.org Copyright © 2018 Cold Spring Harbor Laboratory Press; all rights reserved; doi: 10.1101/cshperspect.a032649 Cite this article as Cold Spring Harb Perspect Biol 2018;10:a032649 1 Downloaded from http://cshperspectives.cshlp.org/ on September 25, 2021 - Published by Cold Spring Harbor Laboratory Press T.E. Dever et al. Aminoacyl-tRNA EF1A EF1A EPA Aminoacyl-tRNA binding eEF1B EF2 EF1A EF1A Deacyl-tRNA EPA Translocation EF2 A-site tRNA accommodation EPA Hypusine eIF5A EPA EF2 Peptide bond Diphthamide formation EPA Figure 1. Model of the eukaryotic translation elongation pathway. At the top, an eEF1A•GTP•aminoacyl-tRNA (transfer RNA) ternary complex binds to the A (aminoacyl) site of an 80S ribosome with the anticodon loop of the tRNA in contact with the messenger RNA (mRNA). Following GTP hydrolysis and release of an eEF1A•GDP binary complex, the aminoacyl-tRNA is accommodated into the A site, and the eEF1A•GDP is recycled to eEF1A•GTP by the exchange factor eEF1B. During catalysis of peptide bond formation, the A- and P (pep- tidyl)-site tRNAs shift into hybrid states with the acceptor ends of the tRNAs moving to the P and E sites, respectively. Substrate positioning for peptide bond formation is aided by binding of the factor eIF5A and its hypusine modification (green) in the E site. Following peptide bond formation, the factor eEF2•GTP with its diphthamide modification (magenta) binds in the A site and promotes translocation of the tRNAs into the canonical P and E sites. Following release of the deacylated tRNA from the E site, the next cycle of elongation commences with binding of the appropriate eEF1A•GTP•aminoacyl-tRNA to the A site. Throughout, GTP is depicted as a green ball and GDP as a red ball; also, the large ribosomal subunit (light blue) is displayed transparently to enable visualization of the tRNAs, factors, and mRNA bound to the decoding center at the interface between the large and small subunits and of tRNAs, interacting with the peptidyl transferase center in the large subunit. Note, however, that the positions of the mRNA, tRNAs, and factors are drawn for clarity and are not meant to specify their exact places on the ribosome. helix h44 residues A1824 and A1825 in mam- bonding (Ogle et al. 2001; Shao et al. 2016; Love- malian (rabbit) ribosomes (A1755 and A1756 in land et al. 2017). Interestingly, when flipped out Saccharomyces cerevisiae and A1492 and A1493 of helix 44 (h44), the residues A1824 and A1825 in Escherichia coli, respectively) as well as the (or A1492 and A1493 in bacteria) interact with residue G626 in rabbit ribosomes (G577 in the first two codon pairs in the codon–antico- S. cerevisiae and G530 in E. coli) interact with don duplex, enabling the +3 position to partic- the minor groove of the codon–anticodon helix ipate in wobble interactions related to the degen- and stabilize A-site tRNA binding by hydrogen eracy of the genetic code (Loveland et al. 2017). 2 Cite this article as Cold Spring Harb Perspect Biol 2018;10:a032649 Downloaded from http://cshperspectives.cshlp.org/ on September 25, 2021 - Published by Cold Spring Harbor Laboratory Press Translation Elongation and Recoding As the h44 residues also flip out to interact with into the A site, peptide bond formation with mispaired codon–anticodon helices formed the peptidyl-tRNA in the P site occurs rapidly. with near-cognate tRNAs in the A site (De- Composed of conserved rRNA elements in the meshkina et al. 2012), it has been proposed large ribosomal subunit, the peptidyl transferase that the interaction of G626 (G530 in bacteria) center (PTC) of the ribosome is well conserved may perform a more crucial function as the between bacteria and eukaryotes (Ben-Shem latching nucleotide that fixes the codon–antico- et al. 2010, 2011; Klinge et al. 2011), and prin- don helix in the decoding center of the ribosome cipally functions by positioning the peptidyl- (Loveland et al. 2017). In addition to providing and aminoacyl-tRNAs for catalysis. The factor insights into decoding, the recent structures of eIF5A, the ortholog of bacterial elongation fac- eukaryotic ribosomal complexes have provided tor P (EF-P), binds in the E site, interacts with insights into GTPase activation of eEF1A as well the acceptor arm of the peptidyl-tRNA, and is as of eRF3 in termination complexes and of thought, like EF-P (Doerfel et al. 2015), to pro- Hbs1 in ribosome rescue complexes (Shao mote peptide bond formation by inducing a fa- et al. 2016). In these structures, interactions be- vorable positioning of the substrates (Gutierrez tween the sarcin-ricin loop of the large ribosom- et al. 2013; Melnikov et al. 2016a,b; Schmidt al subunit and the Switch 2 loop of the GTPase et al. 2016; Shin et al. 2017). domains helps position the catalytic His residue During peptide bond formation, the nascent to promote GTP hydrolysis (Shao et al. 2016). peptide is transferred from the peptidyl-tRNA Moreover, the amino terminus of the eukaryote- in the P site to the amino group of the A-site specific ribosomal protein eS30 becomes or- aminoacyl-tRNA (aa-tRNA) to form a new ex- dered upon cognate codon–anticodon interac- tended peptidyl-tRNA. Peptide bond formation tion in the A site, and a conserved His residue is accompanied by repositioning of the tRNAs inserts into the decoding center to form poten- into hybrid states (Moazed and Noller 1989) tially stabilizing contacts (Shao et al. 2016). and by subunit rotation. Cryo-EM imaging of These novel interactions may contribute to the eukaryotic elongation complexes has revealed reported enhanced accuracy of eukaryotic ver- three states of tRNA binding and subunit rota- sus bacterial elongation (Kramer et al. 2010). tion upon peptide bond formation: in unrotated Finally, the structural studies of the eukaryotic complexes, the newly formed peptidyl-tRNA is elongation complex revealed a conserved bind- in the A site and deacylated tRNA is in the P site; ing site for inhibitors of eEF1A and EF-Tu. The in rotated-1 complexes, the deacylated tRNA translational inhibitor didemnin B, which spe- adopts a hybrid P/E state with the anticodon cifically impairs eukaryotic elongation, was paired with the mRNA in the P site and the found to bind in a cleft between the G domain acceptor arm of the tRNA in the E site, while and domain III of eEF1A in a position that over- the peptidyl-tRNA remains in the classic A site; laps with the binding site of the structurally un- and in the rotated-2 state, the deacylated tRNA related antibiotic kirromycin on EF-Tu (Shao is in the hybrid P/E state and the peptidyl-tRNA et al. 2016). Like kirromycin, didemnin B and is repositioned into a hybrid A/P state with the the functionally related eEF1A inhibitor terna- anticodon paired with mRNA in the A site tin (Carelli et al. 2015) are thought to prevent the and the peptide attached to the acceptor arm structural rotations in eEF1A required for re- in the P site (Budkevich et al.
Details
-
File Typepdf
-
Upload Time-
-
Content LanguagesEnglish
-
Upload UserAnonymous/Not logged-in
-
File Pages20 Page
-
File Size-