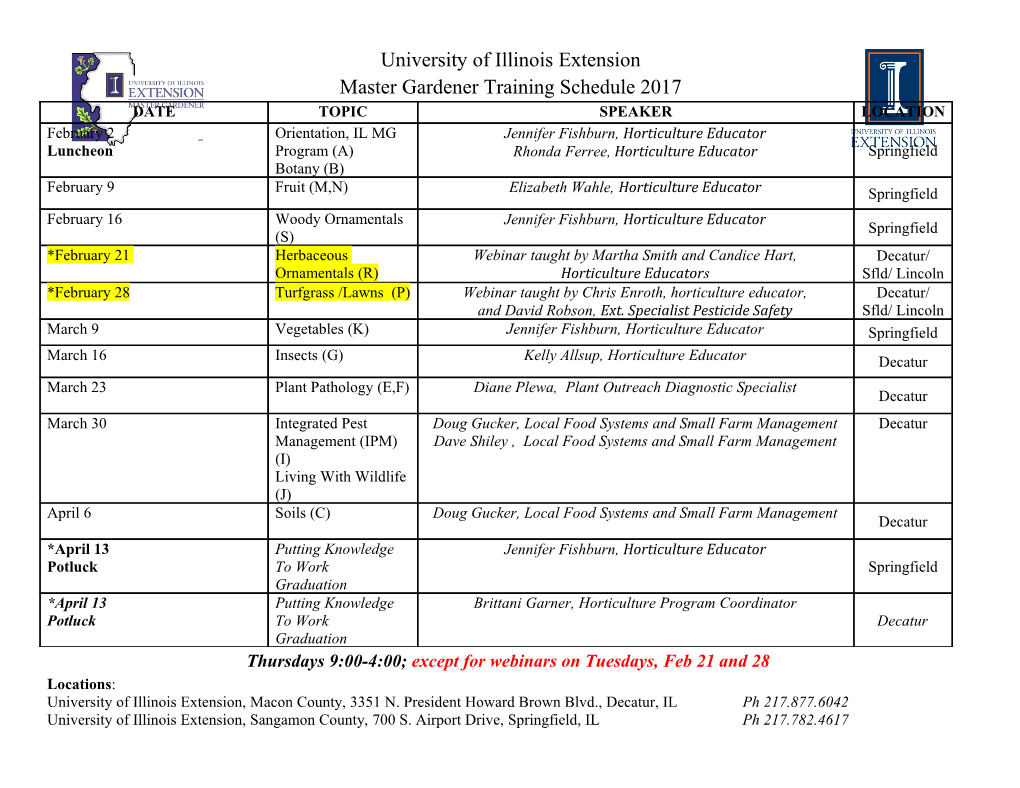
SCALABLE SYNTHESIS OF LITHIUM SULFIDE NANOCRYSTALS FOR NEXT GENERATION BATTERY TECHNOLOGIES by Yangzhi Zhao A thesis submitted to the Faculty and the Board of Trustees of the Colorado School of Mines in partial fulfillment of the requirements for the degree of Doctor of Philosophy (Materials Science) Golden, Colorado Date: Signed: Yangzhi Zhao Signed: Dr. Coiln A. Wolden Thesis Advisor Signed: Dr. Yongan Yang Thesis Advisor Golden, Colorado Date: Signed: Dr. Eric Toberer Professor and HeaD Materials Science Program ii ABSTRACT Batteries play extremely important roles in electricity storage systems. Managing the temporal nature of ever increasing renewable power generation requires next generation batteries with larger power and energy density, safer operation, and lower costs. Lithium sulfide (Li2S) nanocrystals (NCs) are critical materials used to make soliD-state electrolytes and cathodes for emerging battery technologies. Li2S is typically produced by high temperature carbothermal reduction that creates powders, which then require extensive ball milling to produce the nano-sized materials desirable for battery applications. We have recently developed a solution-based synthesis of Li2S by contacting metalorganic solutions with hydrogen sulfide at ambient temperature. This green chemistry proceeds with an atom economy approaching unity with additional benefits that include complete abatement of H2S and recovery of the valuable H2 stored within. Previous work in the group employed an approach where the NCs were directly precipitated out of solution. However, several challenges remain. The resulting NCs were relatively large (>100 nm), limiting their electrochemical activity, and Li2S recovery was only ~85%. In this thesis I developed a modified approach in which Li2S remains fully dissolved in solution, with NCs being recovered in a subsequent evaporation step. Although this introduced an extra processing step, benefits include the ability to increase the concentration and thus the amount of material produced per batch, as well as nominally 100% recovery of the valuable Li reagent. Moreover, control of nanocrystal size and uniformity is demonstrated through choice of solvent and manipulation of processing conditions such as precursor concentration and solvent evaporation rate. X-ray diffraction (XRD), small angle X-ray scattering (SAXS), and scanning electron microscopy (SEM) were used to quantify crystallinity, particle size distribution (PSD), and morphology, respectively. These complementary techniques confirmed the production of anhydrous, phase-pure Li2S nanocrystals with tunable size (5-40 nm) and narrow PSDs. Mild annealing conditions were identified that provide the purity required for battery applications, while retaining the original PSD. Cathodes fabricated using these Li2S NCs obtained 98.5% of theoretical capacity as well as promising cyclability and rate capability, which is the among the best performance reported to date for Li2S cathodes fabricated by simply mixing sulfide with common binder and carbon additives. The process-structure-property-performance relationship in these iii systems has been successfully established, where promising cathode performance stems from ultra-small NCs (< 10 nm) after going through mild annealing at 250°C. The solvo-evaporation process was first demonstrated in a small Parr reactor, where typical yields were ~90 mg/batch. While sufficient for cathode fabrication gram quantities are required for meaningful development of solid state electrolytes (SSE). In this thesis I contributed to scale- up of these processes, including transferring the solvation synthesis step to a scalable bubble column and building a fluidized bed to execute the annealing process. These advances increases our capacity to >1.3 g/batch, enabling the study of solid state electrolyte synthesis. Li2S-P2S5 based glassy electrolytes formed by a combination of ball milling and cold pressing were used to illustrate the benefits of our Li2S NCs. It was shown that Li2S NCs reduced the mechanical ball mixing time required to make Li2S-P2S5 based glasses by at least 60% relative to commercial Li2S micro-powders. 70Li2S-30P2S5 glassy electrolytes were fabricated by colD pressing and characterized by impedance spectroscopy and chronoamperometry. The pellet density increases with hydraulic pressure as expected. Optimum performance was achieved at 300 MPa, which reflected a balance between densification and crack formation. SSEs formed at this condition exhibited high ionic conductivity (~10-5 S cm-1), low electronic conductivity (~10-10 S cm-1), and reduced activation barrier (~ 30 kJ/mol). These properties were comparable to leading reports in the literature, validating the use of our material for solid state electrolyte fabrication. iv TABLE OF CONTENTS ABSTRACT ................................................................................................................................... iii LIST OF FIGURES ....................................................................................................................... xi LIST OF TABLES ....................................................................................................................... xvi LIST OF ABBREVIATIONS ..................................................................................................... xvii ACKNOWLEDGEMENT ......................................................................................................... xviii CHAPTER 1 WHY BEYOND LITHIUM ION BATTERY ......................................................... 1 1.1 Introduction and Motivation ................................................................................................. 1 1.2 Beyond Lithium Ion Batteries ............................................................................................... 3 1.3 Objective of thesis ................................................................................................................. 5 1.4 Reference .............................................................................................................................. 6 CHAPTER 2 LITERATURE REVIEW OF SULFIDE BASED BATTERIES & MATERIALS ................................................................................................................................ 10 2.1 Solid State Electrolytes ....................................................................................................... 10 2.1.1 Li2S-P2S5 glassy electrolyte ......................................................................................... 10 2.1.2 Superionic Conductor Li7P3S11 .................................................................................... 11 2.1.3 Argyrodite (Li6PS5X, X=Cl, Br, I) ............................................................................... 12 2.2 Lithium-Sulfur Batteries ..................................................................................................... 13 2.2.1 Volume Expansion of Cathode During Lithiation (Discharging) ................................ 13 2.2.2 Lithium Dendrite Growth on Anode ............................................................................ 13 2.2.3 Polysulfide Shuttling Effect ......................................................................................... 14 2.3 A Key Material- Li2S Nanocrystals .................................................................................... 15 2.3.1 Why Li2S ...................................................................................................................... 15 2.3.2 Current Synthetic Approach of Metal Sulfides ........................................................... 16 2.4 Reference ............................................................................................................................ 17 CHAPTER 3 METHODOLOGY ................................................................................................ 22 v 3.1 Synthesis Approach ............................................................................................................ 22 3.2 Lab Set Up .......................................................................................................................... 22 3.2.1 Glovebox Rehabilitation .............................................................................................. 23 3.2.2 Purifier Catalyst Replacement ..................................................................................... 24 3.2.3 Ball Mixer .................................................................................................................... 25 3.2.4 Tube Furnace ............................................................................................................... 25 3.2.5 Gamry Cables Feed Through Glovebox ...................................................................... 25 3.2.6 Fluidized Bed Apparatus .............................................................................................. 26 3.3 Experimental Details ........................................................................................................... 27 3.3.1 Pellet Formation By ColD-Pressing ............................................................................. 27 3.3.2 Magnetron Sputtering .................................................................................................. 27 3.3.3 T-Dependence Electrochemical Impedance Spectroscopy (EIS) ................................ 27 3.4 Characterization Technique ...............................................................................................
Details
-
File Typepdf
-
Upload Time-
-
Content LanguagesEnglish
-
Upload UserAnonymous/Not logged-in
-
File Pages180 Page
-
File Size-