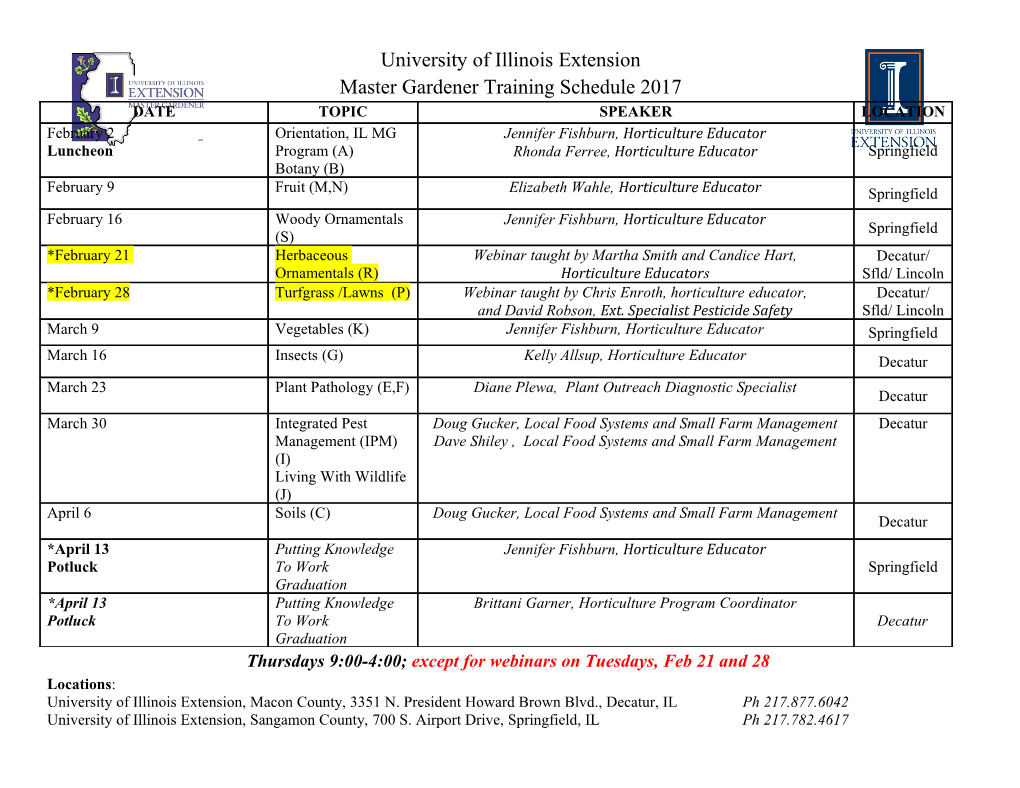
1 Title: New constraints on equatorial temperatures during a Late 2 Neoproterozoic snowball Earth glaciation 3 4 5 Authors: Ryan C. Ewing1*, Ian Eisenman 2, Michael P. Lamb 3, Laura Poppick4, Adam C. 6 Maloof4, Woodward W. Fischer3 7 8 Affiliations: 9 10 1Department of Geology and Geophysics, Texas A&M University, College Station, TX 77843 11 12 2Scripps Institution of Oceanography, University of California at San Diego, La Jolla, CA 92093. 13 14 3Division of Geological and Planetary Sciences, California Institute of Technology, Pasadena, CA 91125. 15 16 4Department of Geosciences, Princeton University, Princeton, NJ 08544. 17 18 * Correspondence to: [email protected] 19 20 21 22 23 1 24 Abstract: 25 26 Intense glaciation during the end of Cryogenian time (~635 million years ago) marks 27 the coldest climate state in Earth history - a time when glacial deposits accumulated 28 at low, tropical paleolatitudes. The leading idea to explain these deposits, the snowball 29 Earth hypothesis, predicts globally frozen surface conditions and subfreezing 30 temperatures, with global climate models placing surface temperatures in the tropics 31 between -20°C and -60°C. However, precise paleosurface temperatures based upon 32 geologic constraints have remained elusive and the global severity of the glaciation 33 undetermined. Here we make new geologic observations of tropical periglacial, 34 aeolian and fluvial sedimentary structures formed during the end-Cryogenian, 35 Marinoan glaciation in South Australia; these observations allow us to constrain 36 ancient surface temperatures. We find periglacial sand wedges and associated 37 deformation suggest that ground temperatures were sufficiently warm to allow for 38 ductile deformation of a sandy regolith. The wide range of deformation structures 39 likely indicate the presence of a paleoactive layer that penetrated 2-4 meters below 40 the ground surface. These observations, paired with a model of ground temperature 41 forced by solar insolation, constrain the local mean annual surface temperature to 42 within a few degrees of freezing. This temperature constraint matches well with our 43 observations of fluvial deposits, which require temperatures sufficiently warm for 44 surface runoff. Although this estimate coincides with one of the coldest near sea-level 45 tropical temperatures in Earth history, if these structures represent peak Marinaon 46 glacial conditions, they do not support the persistent deep freeze of the snowball Earth 2 47 hypothesis. Rather, surface temperatures near 0°C allow for regions of seasonal 48 surface melting, atmosphere-ocean coupling and possible tropical refugia for early 49 metazoans. If instead these structures formed during glacial onset or deglaciation, 50 then they have implications for the timescale and character for the transition into or 51 out of a snowball state. 52 53 1. Introduction: 54 55 Observations of globally-distributed glacial sediments deposited at sea-level in low 56 paleolatitudes (Harland, 2007; Hoffman and Schrag, 2002; Williams, 1975) gave rise to 57 claims that Earth’s late Neoproterozoic glacial climate must have been radically different 58 from that of Phanerozoic glacial intervals (Hoffman and Schrag, 2002; Hoffman et al., 59 1998; Kirschvink, 1992; Williams, 1975). The origin and significance of these glaciogenic 60 deposits has been debated for nearly a century (see review in Harland, 2007), but the 61 snowball Earth hypothesis has emerged as a unifying hypothesis to explain the evidence. 62 The snowball Earth model proposes that Cryogenian climatic events drove equatorial 63 temperatures to below -20°C, temperatures sufficient to freeze Earth’s oceans from pole to 64 equator (Hoffman and Schrag, 2002; Kirschvink, 1992). Variants on the snowball Earth 65 model propose that the equatorial regions of Earth’s oceans remained open and Earth’s 66 surface temperatures, though cold, were not sufficient to freeze the entirety of Earth’s 67 surface (Abbot et al., 2011; Crowley et al., 2001; Peltier et al., 2007). Direct geological 68 constraints on surface temperatures during this period are missing, and evidence supporting 69 any one hypothesis has thus far been equivocal. 3 70 71 Although abundant glacial sediments deposited at low latitudes during Cryogenian time 72 provide the primary evidence for a cold global climate, significant differences in the 73 environment are expected depending on the temperature of Earth’s surface. For example, 74 at the temperatures expected by the end-member model of a snowball Earth, the range of 75 active sedimentary processes during the peak glacial periods would be limited (Allen and 76 Etienne, 2008). Wind-blown sediments may be made unavailable for transport because of 77 ice burial or ice cementation. Temperatures would be too cold for fluvial activity and 78 modification of continental shelf sediments by tidal and wave action would be greatly 79 attenuated (Hoffman and Schrag, 2002). Models that do not require deeply frozen 80 temperatures allow a wider range of sedimentary environments associated with an active 81 hydrologic cycle and open oceans to be active throughout the glaciation and, in certain 82 localities, such sedimentary environments have been highlighted as counter evidence to the 83 presence of a snowball Earth during the Cryogenian (Allen and Etienne, 2008). 84 85 Periglacial sand wedges and associated regolith deformation and fluvial deposits found in 86 the Marinoan Whyalla Sandstone in South Australia, are a primary focus of this study (Fig. 87 1). This suite of sedimentary structures forms under a relatively narrow thermal regime 88 (Mellon, 1997; Pewe, 1959) and can be used to refine equatorial temperature estimates 89 during the Marinoan glaciation. Models and modern observations of sand wedges indicate 90 that wedges form by sediment infilling of thermal contraction cracks, which occur when 91 the ground cools and induces a tensile stress that exceeds the strength of frozen regolith 92 (Maloof et al., 2002; Mellon, 1997; Pewe, 1959). Large seasonal temperature variations 4 93 found at high latitudes, very cold ground (mean annual temperature < 0ºC), and a dry 94 climate are key characteristics of these models and observations that indicate crack and fill 95 cycles that give rise to vertically laminated, sand-filled wedges, which may be associated 96 with deformed ground (Fig. 2). (Black, 1976; Hallet et al., 2011; Pewe, 1959; Sletten et al., 97 2003). Deformed ground associated with sand wedge growth occurs because sand fills the 98 open fracture and during a warm phase of the cycle the ground expands; the fracture cannot 99 close and compressive stresses propagate horizontally to deform the ground surrounding 100 the wedge (Hallet et al., 2011). 101 102 Some of these structures have long presented a conundrum for reconstructing Earth’s 103 equatorial climate during the Marinoan glaciation in South Australia. The presence of sand 104 wedges in particular, because they are thought to require a strong seasonal temperature 105 variation, are difficult to explain at equatorial latitudes where seasonal temperature 106 variations are low. Their presence in the low latitudes, along with low-latitude glacial 107 deposits, inspired the proposal that Earth’s obliquity was higher prior to the Cambrian 108 Period (Williams, 1975; Williams, 2007). An alternative hypothesis suggested that the 109 wedges formed by diurnal temperature variations under severely cold equatorial conditions, 110 consistent with the snowball Earth hypothesis (Maloof et al., 2002). 111 112 Here we revisit the origin of these sand wedges in the context of other co-existing 113 sedimentary structures and paleoenvironments. In order to explain these features and place 114 constraints on the paleotemperatures during the Marinoan Glaciation in South Australia, 115 we pair geologic observations with a model of ground temperatures forced by solar 5 116 insolation. The model provides a better understanding of the expected temperature change 117 within which low-paleolatitude sedimentary deposits develop, and it is used along with 118 observations of periglacial sand wedges to constrain temperatures. Observations of an 119 active fluvial system corroborate the primary conclusion of this work that temperatures 120 during the Marinoan glaciation in South Australia were warmer than anticipated by a 121 snowball Earth and were likely near 0°C. 122 123 2. Geologic Background 124 125 The Cryogenian Whyalla Sandstone and Cattle Grid Breccia contain abundant sand wedges, 126 periglacial deformation structures, and aeolian and fluvial deposits. These deposits sit on 127 the Stuart Shelf, which is adjacent to the Adelaide Rift Complex (ARC) (Preiss, 1987; 128 Williams and Tonkin, 1985; Williams, 1998). During Late Neoproterozoic time, the Stuart 129 Shelf and ARC were part of a broad continental margin undergoing episodes of rifting and 130 thermal subsidence, which provided accommodation for a 7-12 km succession of 131 Neoproterozoic to Cambrian deposits, directly overlying Paleo- and Mesoproterozoic 132 basement (Preiss, 1987). The relatively undeformed Stuart Shelf lies to the west of the 133 ARC and preserves thin, Neoproterozoic to Cambrian cratonic cover that onlaps the Gawler 134 Craton (Fig. 1). 135 136 2.1 Stratigraphy 137 6 138 The Whyalla Sandstone is part of the Umberatana Group, which is the primary Cryogenian 139 glaciogenic sedimentary succession in South Australia. The Whyalla Sandstone is 140 interpreted as
Details
-
File Typepdf
-
Upload Time-
-
Content LanguagesEnglish
-
Upload UserAnonymous/Not logged-in
-
File Pages35 Page
-
File Size-