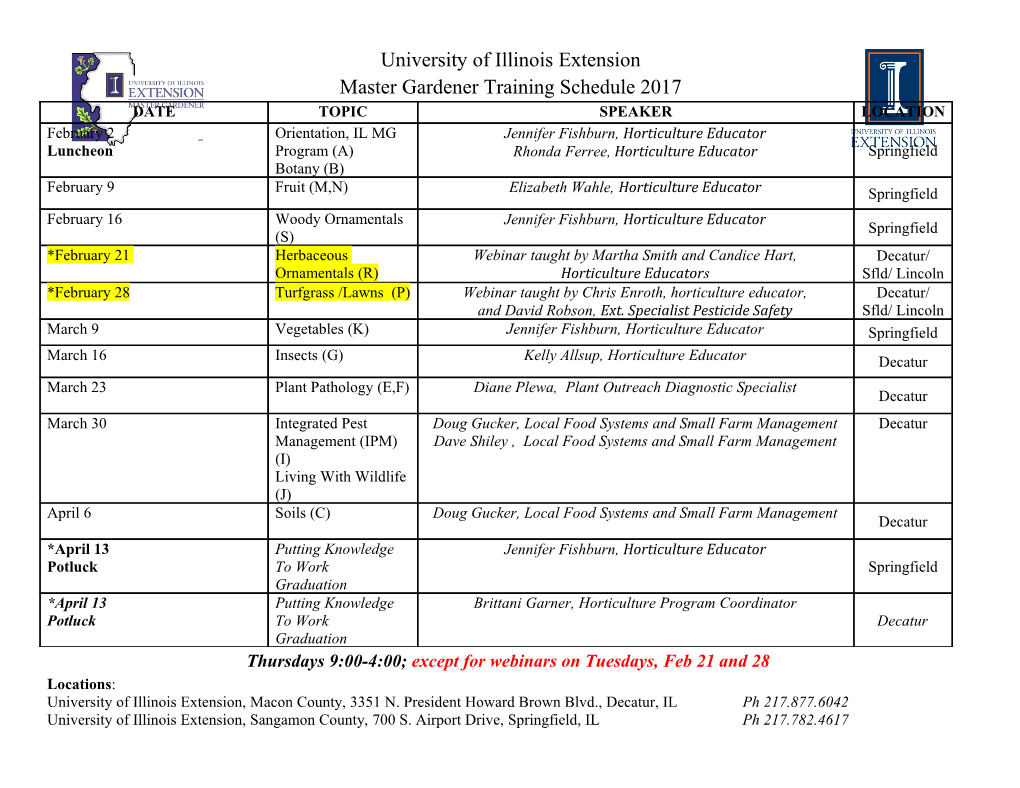
Lee et al. Microb Cell Fact (2017) 16:39 DOI 10.1186/s12934-017-0646-4 Microbial Cell Factories REVIEW Open Access Cellular factories for coenzyme Q10 production Sean Qiu En Lee1, Tsu Soo Tan5, Makoto Kawamukai6 and Ee Sin Chen1,2,3,4* Abstract Coenzyme Q10 (CoQ10), a benzoquinone present in most organisms, plays an important role in the electron-transport chain, and its deficiency is associated with various neuropathies and muscular disorders. CoQ10 is the only lipid- soluble antioxidant found in humans, and for this, it is gaining popularity in the cosmetic and healthcare industries. To meet the growing demand for CoQ10, there has been considerable interest in ways to enhance its production, the most effective of which remains microbial fermentation. Previous attempts to increase CoQ10 production to an indus- trial scale have thus far conformed to the strategies used in typical metabolic engineering endeavors. However, the emergence of new tools in the expanding field of synthetic biology has provided a suite of possibilities that extend beyond the traditional modes of metabolic engineering. In this review, we cover the various strategies currently undertaken to upscale CoQ10 production, and discuss some of the potential novel areas for future research. Keywords: Coenzyme Q10, Isoprenoid, Antioxidant, Industrial biosynthesis, Protein engineering, Synthetic biology Background various aspects of CoQ biosynthesis have been described Coenzyme Q, commonly known as ubiquinone or CoQ, at length elsewhere [1–6]. is a lipid-soluble, powerful antioxidant, and an essential CoQ10 is the only lipid-soluble antioxidant produced cofactor in mitochondrial oxidative phosphorylation [1– by humans, and it localizes to almost every membrane, 3]. Coenzyme Q is species specific, with differences dic- ranging from mitochondrial membranes to that of very tated by the number of isoprenyl units on the isoprenoid low density lipoproteins (VLDL) [7]. This solubility side chain. For example, 10 isoprenyl units are found in means that CoQ can protect lipoproteins and lipids from human and the fission yeast Schizosaccharomyces pombe peroxidation and oxidative damage [8]. CoQ10 also serves but fewer units are found in other species (CoQ8 in alongside other antioxidants, such as vitamins C and E, Escherichia coli, CoQ9 in Arabidopsis thaliana, and CoQ6 to combat free-radical damage arising from energetic in Saccharomyces cerevisiae) [1]. The isoprenoid side mitochondrial reactions [9, 10]. Given its myriad func- chain is responsible for the lipid-soluble nature of CoQ, tions and physiological importance, it is not surprising whereas its antioxidant capacity derives from its quinone that CoQ deficiency can result in numerous diseases. head, which can enable electron transfer (Fig. 1). Because In model organisms, such as S. cerevisiae and S. pombe, of this electron-sequestering property, CoQ10 acts as an CoQ deficiency is not lethal but results in growth defects antioxidant at cellular membranes to counteract the oxi- on minimum medium, and a heightened sensitivity to dation of lipids or lipoproteins [4]. CoQ10 has roles in oxidative stress [11–15]. In Caenorhabditis elegans, CoQ other physiological processes, including sulfide oxidation, deficiency leads to GABA neuron degeneration, and in regulating the mitochondrial permeability transition Drosophila melanogaster, it can cause mitochondrial 2 pore, and in the translocation of protons and Ca + across stress and neuronal apoptosis [16, 17]. In humans, CoQ10 biological membranes [5, 6]. A detailed account of the deficiency has been implicated in various diseases involv- ing muscle and neural development, with the severity of *Correspondence: [email protected] the disease correlated with the acuteness of the CoQ10 1 Department of Biochemistry, National University of Singapore, shortfall [18]. These diseases may manifest in condi- Singapore, Singapore tions such as central nervous system (CNS) dysfunction, Full list of author information is available at the end of the article © The Author(s) 2017. This article is distributed under the terms of the Creative Commons Attribution 4.0 International License (http://creativecommons.org/licenses/by/4.0/), which permits unrestricted use, distribution, and reproduction in any medium, provided you give appropriate credit to the original author(s) and the source, provide a link to the Creative Commons license, and indicate if changes were made. The Creative Commons Public Domain Dedication waiver (http://creativecommons.org/ publicdomain/zero/1.0/) applies to the data made available in this article, unless otherwise stated. Lee et al. Microb Cell Fact (2017) 16:39 Page 2 of 16 Frederick Crane first isolated CoQ10 from a bovine heart source in the late 1950s [43]. Since then, industrial attempts to produce CoQ10 have centered on animal tis- sue extraction, semi-chemical synthesis, and microbial fermentation [44, 45]. The chemical synthesis of CoQ10 has typically involved Fig. 1 Chemical structure of coenzyme Q10. This molecule consists of a isoprenoid side chain composed of ten tandemly linked isoprenyl solanesol as a starting substrate and the source of the groups attached to a quinone head group isoprenoid tail, and this is carried out before it is com- bined with the quinone head [46]. However, as with most chemical processes, there are numerous costs associ- myopathy or cardiomyopathy, among others [19–22]. The ated with such high-energy catalysis reactions because oxidative damage associated with impaired CoQ10 func- of the need for expensive substrates and because of the tion has also been implicated in numerous clinical phe- significant chemical waste generated from its produc- notypes [18, 23–25]. tion [47–49]. The chemical synthesis of CoQ10 also lacks By virtue of its therapeutic relevance, CoQ10 is of par- stereoselectivity, and this makes it difficult to separate ticular importance in the biomedical and health sup- optical isomers to obtain the all-trans biologically viable plement scene. Oral CoQ10 supplements are often isomer [50]. prescribed alongside treatments for various diseases [26]. Owing to these difficulties, microbial biosynthesis has One example is its co-administration with HMG-CoA become a preferred avenue of CoQ10 production. The (3-hydroxy-3-methylglutaryl-coenzyme A) reductase cell-based catalysis of compounds does not require harsh (HMGR) inhibitors, widely used cholesterol-lowering catalytic conditions of heat and pressure that typify many drugs otherwise known as statins. HMGR catalyzes the chemical synthesis processes. Furthermore, the produc- formation of mevalonic acid, the precursor for choles- tion costs tend to be lower, cheap growth media provides terol and CoQ10 biosyntheses [27]. Patients using statins an appropriate substrate, and expensive co-substrates can show lower blood levels of CoQ10, and this justifies the be recycled [48, 51]. A living cellular system is also scal- need for CoQ10 supplementation to reduce the cardio- able, and the precision of the cellular catalytic machinery myopathy risk associated with statin use [27–30]. The circumvents the problems of stereoselectivity [52, 53]. presence of CoQ10 is however implicated in resistance Furthermore, unlike with chemical processing, altered to chemotherapeutic drugs, and this calls for caution in genetics does not significantly affect the operating costs, administering CoQ10 alongside certain agents [31, 32]. meaning that the efforts associated with constructing a CoQ10 production decreases with aging [33], as does high-titer-producing organism are worthwhile. Through the antioxidant capability of the cell. Increased oxida- microbial biosynthesis, metabolic engineering approaches tive stress in aging cells may be ameliorated with dietary can be used to increase the titer of CoQ10 and overcome supplementation of CoQ10 [34]. Indeed, CoQ10 has gar- some of the limiting steps along the biosynthetic pathway. nered great popularity as an antioxidant in moisturizers, Metabolic engineering approaches initially used chemi- anti-wrinkle and anti-aging skin care treatments [35–37]. cal mutagenesis-based selection and chemical engineer- With the growing demand for skin care cosmetics and ing procedures that centered on manipulating substrate public awareness of the importance of antioxidants, we flux; however, the field has since expanded to include will likely see an increase in the demand for CoQ10 prod- other strategies from a genetics standpoint [48, 54]. ucts on the market quite quickly [38]. Given that CoQ10 The process varies depending on promoter choice and is endogenously synthesized, there should be fewer strength, cassette copy number, and the localization or unwanted side effects from its therapeutic use as com- tethering of enzymes to scaffolds [55, 56]. The choice pared with other synthetic compounds, and this has been of cassette and promoter are typically host depend- supported by tolerability studies for high CoQ10 doses ent, given that promoter strength and usability rely on a [39]. Hence, attention has surged in the therapeutic use species-specific genetic environment and functionality. of CoQ10 in non-curable diseases challenging modern Furthermore, enzymes involved in the tail end of CoQ10 societies including Alzheimer’s, Huntington’s and Parkin- production are localized in the mitochondria, leading to son’s, and cardiovascular diseases [40–42]. models that propose the involvement of a membrane- bound complex containing multiple polypeptides of the Industrial
Details
-
File Typepdf
-
Upload Time-
-
Content LanguagesEnglish
-
Upload UserAnonymous/Not logged-in
-
File Pages16 Page
-
File Size-