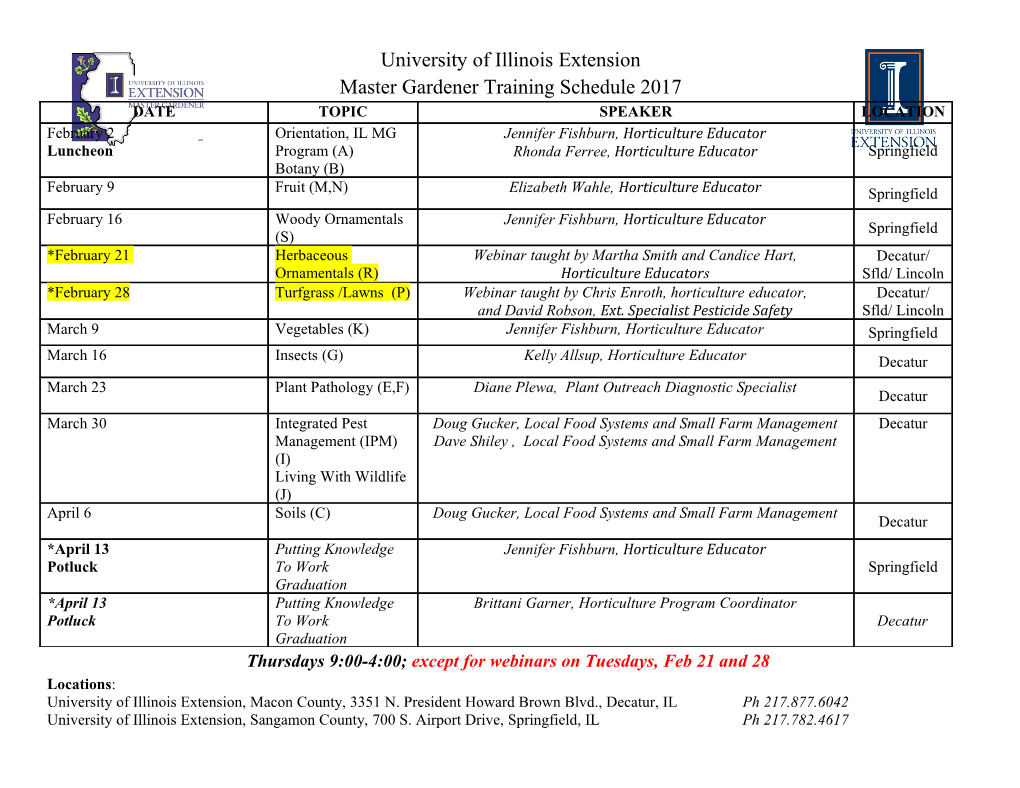
The Cryosphere, 10, 1707–1719, 2016 www.the-cryosphere.net/10/1707/2016/ doi:10.5194/tc-10-1707-2016 © Author(s) 2016. CC Attribution 3.0 License. MABEL photon-counting laser altimetry data in Alaska for ICESat-2 simulations and development Kelly M. Brunt1,2, Thomas A. Neumann2, Jason M. Amundson3, Jeffrey L. Kavanaugh4, Mahsa S. Moussavi5,6, Kaitlin M. Walsh2,7, William B. Cook2, and Thorsten Markus2 1Earth System Science Interdisciplinary Center (ESSIC), University of Maryland, College Park, MD, USA 2NASA Goddard Space Flight Center, Greenbelt, MD, USA 3Department of Natural Sciences, University of Alaska Southeast, Juneau, AK, USA 4Department of Earth and Atmospheric Sciences, University of Alberta, Edmonton, AB, Canada 5Cooperative Institute for Research in Environmental Sciences (CIRES), University of Colorado, Boulder, CO, USA 6National Snow and Ice Data Center (NSIDC), CIRES, University of Colorado, Boulder, CO, USA 7Stinger Ghaffarian Technologies, Inc., Greenbelt, MD, USA Correspondence to: Kelly M. Brunt ([email protected]) Received: 10 December 2015 – Published in The Cryosphere Discuss.: 19 January 2016 Revised: 1 July 2016 – Accepted: 11 July 2016 – Published: 10 August 2016 Abstract. Ice, Cloud, and land Elevation Satellite-2 (ICESat- and melt ponds indicate the potential ICESat-2 will have for 2) is scheduled to launch in late 2017 and will carry the the study of mountain and other small glaciers. Advanced Topographic Laser Altimeter System (ATLAS), which is a photon-counting laser altimeter and represents a new approach to satellite determination of surface ele- vation. Given the new technology of ATLAS, an airborne 1 Introduction instrument, the Multiple Altimeter Beam Experimental Li- dar (MABEL), was developed to provide data needed for Ice, Cloud, and land Elevation Satellite-2 (ICESat-2) is a satellite-algorithm development and ICESat-2 error analysis. NASA mission scheduled to launch in 2017. ICESat-2 is MABEL was deployed out of Fairbanks, Alaska, in July 2014 a follow-on mission to ICESat (2003–2009) and will ex- to provide a test dataset for algorithm development in sum- tend the time series of elevation-change measurements aimed mer conditions with water-saturated snow and ice surfaces. at estimating the contribution of polar ice sheets to eu- Here we compare MABEL lidar data to in situ observa- static sea level rise. ICESat-2 will carry the Advanced To- tions in Southeast Alaska to assess instrument performance pographic Laser Altimeter System (ATLAS), which uses in summer conditions and in the presence of glacier surface a different surface detection strategy than the instrument melt ponds and a wet snowpack. Results indicate the follow- onboard ICESat. Abdalati et al. (2010) provide an early ing: (1) based on MABEL and in situ data comparisons, the overview of the ATLAS concept and overall design. While ATLAS 90 m beam-spacing strategy will provide a valid as- the measurement goals of ATLAS remain as described in sessment of across-track slope that is consistent with shal- Abdalati et al. (2010), some of the details have evolved low slopes (< 1◦) of an ice-sheet interior over 50 to 150 m (Markus et al., 2016). ATLAS is a six-beam, photon-counting length scales; (2) the dense along-track sampling strategy laser altimeter (Fig. 1). In a photon-counting system, single- of photon counting systems can provide crevasse detail; and photon sensitive detectors are used to record arrival time of (3) MABEL 532 nm wavelength light may sample both the any detected photon. ATLAS will use short (< 2 ns) 532 nm surface and subsurface of shallow (approximately 2 m deep) (green) wavelength laser pulses, with a 10 kHz repetition supraglacial melt ponds. The data associated with crevasses rate, which yields a ∼ 0.70 m along-track sampling interval, and a ∼ 17 m diameter footprint. An accurate assessment of ice-sheet surface-elevation change based on altimetry is de- Published by Copernicus Publications on behalf of the European Geosciences Union. 1708 K. M. Brunt et al.: MABEL photon-counting laser altimetry data in Alaska (July 2014) to collect data, including from glacier targets, and to assess elements of the resulting data that may vary seasonally. The Greenland 2012 campaign sampled winter- ICESat-2 (2017) MABEL like conditions, while the Alaska 2014 campaign was timed to collect data during the summer melt season, which is char- ~ ~ acterized by open crevasses and surface melt ponds. In win- ~ ~500 km ~ ter, increased albedo, reduced ice-sheet surface roughness, ~20 km and reduced solar background and backscatter in the atmo- ~3 km ~0.2 km (AK) ~90 m ~2 km max sphere all lead to an increased signal-to-noise ratio and an increase in photon-retrieval density (i.e., the number of, and temporal distribution of photons transmitted and recorded by the lidar). In general, with increased photon-retrieval density, we expect better surface measurement precision. In the ex- Figure 1. Schematic ICESat-2 and MABEL beam geometry treme case, the photon-retrieval density may be sufficiently (dashed lines) and reference ground tracks (grey lines along ice- high that the instrument receiver does not have the time re- sheet surface). ICESat-2 beam pairs (separated by ∼ 90 m) do not have the same energy in order to keep the required laser energy low; quired to process the incoming photon information before therefore, each beam pair consists of a strong and a weak beam receiving more. This effect is referred to as “instrument dead (as indicated by the dash difference). MABEL allows for beam- time” and can produce a positive surface elevation bias. In geometry changes with a maximum ground spacing of ∼ 2 km summer, reduced albedo, increased ice-sheet surface rough- at 20 km. However, for the 2014 AK deployment, the maximum ness, and increased solar background leads to a decrease in ground spacing was 0.2 km (after Brunt et al., 2014). photon-retrieval density and signal-to-noise ratios, compro- mising measurement precision. The Alaska 2014 campaign also aimed to investigate how light at 532 and 1064 nm wave- pendent upon knowledge of local slope (Zwally et al., 2011). lengths interacts with the surface in melting conditions, and Therefore, the six ATLAS beams are arranged into three sets how this may affect the statistics of the 532 nm signal pho- of pairs. Spacing between the three pair sets is ∼ 3 km to in- tons and overall elevation accuracy. crease sampling density, while spacing between each beam Here, we compare in situ measurements with MABEL within a given pair will be ∼ 90 m to make the critical de- airborne lidar data on the Bagley (16 July 2014; 60.5◦ N, termination of local slope on each pass. Therefore, elevation 141.7◦ W) and Juneau (31 July 2014; 58.6◦ N, 134.2◦ W) change can be determined from only two passes of a given icefields in Southeast Alaska (Fig. 2). These comparisons area (Brunt et al., 2014). are made with consideration for the planned ATLAS beam Given this new approach to satellite surface elevation geometry in order to investigate instrument performance in measurement, an airborne instrument, the Multiple Altime- summer conditions and in the presence of surface crevasses ter Beam Experimental Lidar (MABEL), was developed to and melt ponds. (1) enable the development of ICESat-2 geophysical algo- rithms prior to launch and (2) enable ICESat-2 error analy- sis. MABEL (discussed in detail in McGill et al., 2013) is 2 Data and methods a multibeam, photon-counting lidar, sampling at both 532 (green) and 1064 nm (near infrared) wavelengths using short 2.1 MABEL data (∼ 1.5 ns) laser pulses. The dual wavelength instrument de- sign was intended to assess green-wavelength light pene- MABEL data (Level 2A, release 9) for the Alaska tration in water or snow (McGill et al., 2013). Deems et 2014 campaign (Fig. 2) are available from the NASA al. (2013) provide a review of lidar use for snow studies and ICESat-2 website (http://icesat.gsfc.nasa.gov/icesat2/data/ describe how light at 532 and 1064 nm wavelengths interacts mabel/mabel_docs). Each data file contains 1 min of data with snow surfaces. Light penetration into a snow surface for every available beam (approximately two beams per de- is a function of both grain size (with larger snow-grain size ployment were compromised due to instrumentation issues). resulting in increased volumetric scattering, and therefore The data files contain photon arrival times resulting from increased light penetration) and wavelength (with 532 nm reflected laser light (i.e., signal photons), solar background light having lower absorption than 1064 nm light, which ul- and backscatter in the atmosphere (i.e., background photons), timately produces increased light penetration at the shorter and to a lesser degree detector noise (i.e., noise photons). wavelength). Deems et al. (2013) also note that light pene- A histogram-based surface-finding algorithm developed at tration into snow surfaces is extremely difficult to accurately NASA Goddard Space Flight Center (GSFC) was used to measure. discriminate signal photons from background and noise pho- Following engineering test flights in 2010 and 2011, MA- tons. The algorithm is based on histograms of photon arrival BEL was deployed to Greenland (April 2012) and Alaska times in 25 m along-track segments and 10 m vertical bins The Cryosphere, 10, 1707–1719, 2016 www.the-cryosphere.net/10/1707/2016/ K. M. Brunt et al.: MABEL photon-counting laser altimetry data in Alaska 1709 (Fig. 1). The spacing between the individual beams was con- figured to allow simulation of the planned beam geometry of ATLAS. Previous results from the MABEL 2012 Greenland campaign suggest that the ATLAS beam geometry is appro- priate for the determination of slope on ∼ 90 m across-track length scales, a measurement that will be fundamental to ac- counting for the effects of local surface slope from the ice- sheet surface-elevation change derived from ATLAS (Brunt et al., 2014).
Details
-
File Typepdf
-
Upload Time-
-
Content LanguagesEnglish
-
Upload UserAnonymous/Not logged-in
-
File Pages13 Page
-
File Size-