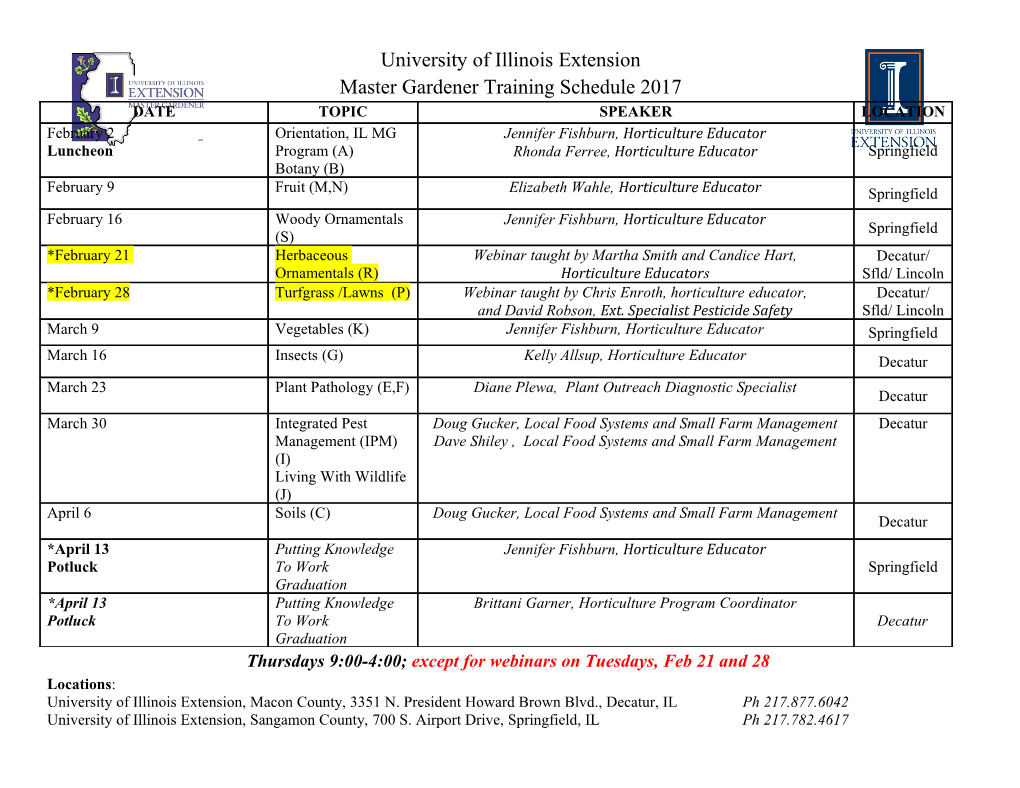
© 2006 Felisa Lauren Wolfe-Simon ALL RIGHTS RESERVED THE ROLE AND EVOLUTION OF SUPEROXIDE DISMUTASES IN ALGAE by FELISA LAUREN WOLFE-SIMON A Dissertation submitted to the Graduate School-New Brunswick Rutgers, The State University of New Jersey In partial fulfillment of the requirements for the degree of Doctor of Philosophy Graduate Program in Oceanography written under the direction of Paul Falkowski and Oscar Schofield and approved by Paul Falkowski Oscar Schofield Jody Hey John Reinfelder Costantino Vetriani Edward Stiefel New Brunswick, New Jersey May 2006 ABSTRACT OF THE DISSERTATION THE ROLE AND EVOLUTION OF SUPEROXIDE DISMUTASES IN ALGAE By FELISA LAUREN WOLFE-SIMON Dissertation Directors: Paul Falkowski and Oscar Schofield Superoxide is a natural byproduct of normal cellular functions and is an important molecule to detoxify because it can obliterate key cellular components. Superoxide .- dismutases catalyze the destruction of the superoxide radical (O2 ) into molecular oxygen and hydrogen peroxide. There are four known varieties of SODs distinguished by their metal cofactor. These are the iron (Fe), manganese (Mn), copper-zinc (CuZn) and nickel (Ni) forms. This enzyme plays a particularly important role in photosynthetic eukaryotes because they have two sources of radical oxygen: photosynthesis and respiration. This dissertation focuses on aspects of SODs in marine secondary red plastid derived algae. Photosynthetic eukaryotes evolved from a singular primary endosymbiosis where a heterotrophic cell engulf and enslaved a cyanobacterium-like plastid. These primary endosymbiotic eukaryotes diverged into the green and red plastid lineages. The secondary red plastid containing algae are the most successful in the modern ocean. Typically, photosynthetic eukaryotes have FeSOD within the plastid. However, laboratory results suggest that the secondary red alga diatom, Thalassiosira ii pseudonana, posses an MnSOD in the plastid which can account for approximately 20% of the total Mn in a cell. This helps close the Mn budget for diatoms. This is a unique use for MnSOD and may contribute to the success of T. pseudonana in the Fe poor modern ocean. This dissertation also addresses the molecular phylogeny of the Fe- and MnSOD family to further understand the extant physiological expression of this enzyme and also examine the phylogenetic position of secondary red algae with respect to the SODs. Because these enzymes are localized with in organelles the genes have likely derived from the original organelle endosymbiont. These data suggest that for all plastid derived eukaryotes FeSOD evolved from the primary endosymbiotic event and was transferred to the nucleus from the plastid because this is the last common ancestor of all these organisms. This includes currently amitochondrial or aplastidic parasites that have subsequently lost these organelles. The MnSOD sequences show monophyly for primary and secondary red algae with a basal relationship to the primary green alga. Because green plants cluster separately with the cyanobacteria, this suggests that the MnSOD of primary green, primary and secondary red plastid eukaryotes evolved from the primary endosymbiotic host cell (i.e. last common ancestor for these groups) and the green plants derived MnSOD from the primary endosymbiont plastid. iii ACKNOWLEDGEMENTS There are so many people I owe a great deal of thanks to that I will just try and start from the beginning. Fundamental to my pursuit of a Ph.D. and was the influence of Dr. Gail Tucker-Griffith as my Advance Placement Biology teacher in high school. It was no small feat to teach this high level course at a performing arts high school! Dr. David Egloff was my undergraduate advisor who anticipated my foray into ocean sciences and algae so much so that he made sure to send me off to the jungles of Costa Rica to study Howler Monkeys. Just to make sure I knew what I wanted to do. I learned how to look up that summer- thanks. I also want to thank Dr. Ivan Valiela for turning me on to biogeochemistry as an undergraduate during a summer REU in Woods Hole. Graduate school has been a tremendously intense learning experience and with out the support and guidance of my co-advisors: Dr. Paul Falkowski and Dr. Oscar Schofield this would never have been possible. Paul for being…Paul; a stimulating, extraordinary, and never predictable challenge. Oscar, for a warm-fuzzy sort of shot in the arm. I also want to thank my committee members for dealing with the interdisciplinary nature of both my project and myself. Drs. John Reinfelder, Costa Vetriani, Jody Hey, and Ed Stiefel. You all always made time for my questions and dire need for feedback. Thank you. Furthermore, I want to especially thank the financial support of the National Science Foundation and Rutgers University. Of course, my fellow graduate students at IMCS are also integral to my success. Jason Sylvan and Grant Law - rock on. Steve Litvin, Tracy Wiegner, Tuo Shi, Sindia Sosdian, Alex Kahl, Carrie Fraser, Steve Tuorto, Rachel Sipler, and others. Thanks for being around when I most needed you! Also, I wish to thank the many post-docs that iv have been through the Falkowski lab. I owe a huge amount of thanks to Drs. Yi-Bu Chen, Yi Sun, Daniel Grzebyk, Michal Koblizek, Diana Nemergut, Lin Jiang, and Ilana Berman-Frank. And of course, a great big thank you hug to Liti Haramaty and Kevin Wyman who put up with my need for constant instrument refresher courses! Finally, my deepest gratitude to my besheert, Jonathan. Without whom none of this would have even been a thought. v TABLE OF CONTENTS ABSTRACT OF THE DISSERTATION ii ACKNOWLEDGEMENTS iv LIST OF TABLES ix LIST OF ILLUSTRATIONS x 1.0 Introduction 1 1.1 Sources and Sinks of ROS in Algae 5 1.2 The SOD Defense System 9 1.2.1 Iron and Manganese SODs 10 1.2.2 Copper-Zinc SODs 12 1.2.3 Nickel SODs 16 1.3 Objectives of Dissertation Research 16 2.0 Review of SODs in Prokaryotic and Eukaryotic Algae 22 2.1 Environmental Regulation of SODs in Algae 23 2.1.1 Visible Light Stress 23 2.1.2. Ultraviolet Radiation 24 2.1.3 Nutrient Stress 25 2.1.4 Metal Toxicity 25 2.2 Algae SODs in an Evolutionary Context 26 2.3 Conclusions and Future Directions 31 vi 3.0 Localization and Role of Manganese Superoxide Dismutase in a Marine Diatom 34 3.1 Introduction 35 3.2 Materials and Methods 37 3.2.1 Organisms, Culture Conditions, and Standard Protocols 37 3.2.2 Cloning and Purification of Recombinant TpMnSOD 37 3.2.3 Antibody Production Against TpMnSOD 38 3.2.4 Immunoblot Analyses 38 3.2.5 Immunogold Staining 39 3.3 Results 41 3.3.1 Native Molecular Mass and Western Analyses 41 3.3.2 MnSOD and the Cellular Manganese Budget in Diatoms 41 3.3.3 Immunolocalization of MnSOD in Plastids 45 3.3.4 Impact of Light on TpMnSOD Expression 45 3.4 Discussion 52 4.0 The Phylogenetic Position of Iron and Manganese Superoxide Dismutases in Secondary Red Algae 58 4.1 Introduction 60 4.2 Materials and Methods 63 4.3 Results 65 4.3.1 FeSOD Clade 70 vii 4.3.2 MnSOD Clade 75 4.3.3 Phototroph Phylogeny 78 4.4 Discussion 80 4.4.1 Position of the primary and secondary plastid-containing eukaryotes 80 4.4.2 The secondary red-plastid puzzle 82 4.4.3 Evidence for LGT beyond endosymbiosis 84 4.4.4 Correlative relationship between the molecular evolution of Photosystems I and II and the Fe/MnSOD family 85 4.5 Conclusion 87 References 95 Curriculum Vita 109 viii LIST OF TABLES Table 1.1: The four one-electron reactions for the reduction of O2 to H2O in aqueous solution and the corresponding reduction potentials. 20 Table 1.2: The basic superoxide dismutase ping-pong mechanism. 21 Table 3.1: Antibody cross reactivity. 56 Table 3.2: Statistics for MnSOD in Thalassiosira pseudonana CCMP1335 57 Table 4.1 All Fe- and MnSOD amino acid sequences used in analyses and their ID 89 Table. 4.2 Averages of similarity indices of Fe- and Mn Superoxide Dismutases. 93 Table 4.3 Prokaryotic and eukaryotic plastid-related sequences used in analyses. 94 ix LIST OF ILLUSTRATIONS Figure 1.1: Seasonal hydrogen peroxide and chlorophyll at the Hawaii Ocean Times Series (HOT) station ALOHA. Data collected from Jan 1994 to Dec 1998 (Gasc et al., 2002). Color represents average chlorophyll in ng•L-1 and contour lines are of equal hydrogen peroxide concentrations in nM. Within the water column algae are the most prominent source of ROS. Significant abiological ROS production would require the presence of free transition metals. At this location, the average trace metal concentrations are too low to produce (from Fenton reactions, see text) the level of ROS observed. Some work has suggested that in coastal and estuarine regions, CDOM may serve as both a source and a sink for ROS {Andrews, 2000 #432;Voelker, 2000 #431;Zepp, 1992 #430;Blough, 1995 #433}, Andrews et al. 2000, Voelker et al. 2000). However, the low nutrient- low chlorophyll waters at the HOT station are unlikely to experience the same levels of CDOM and abiotically produced ROS. 3 Figure 1.2: Synthetic distribution based on known evidence from biochemical and genetic data of the various superoxide dismutases over the tree of life (figure modified after Baldauf et al., 2004). All the potential routes for genetic inheritance are evident in SOD genes. FeSOD is widely distributed between all major clades while specifically MnSOD is more prevalent in Bacteria and Eukaryota. Fe and MnSOD have contrasting potential evolutionary histories. In eukaryotic photosynthetic autotrophs, the ancestral origin of FeSOD may be from x the GSB ancestor of PSI in cyanobacteria, while MnSOD has a variety of possible origins mainly the proteobacterial ancestor to PSII and mitochondria; both plastid metalloforms having been acquired through cyanobacteria.
Details
-
File Typepdf
-
Upload Time-
-
Content LanguagesEnglish
-
Upload UserAnonymous/Not logged-in
-
File Pages132 Page
-
File Size-