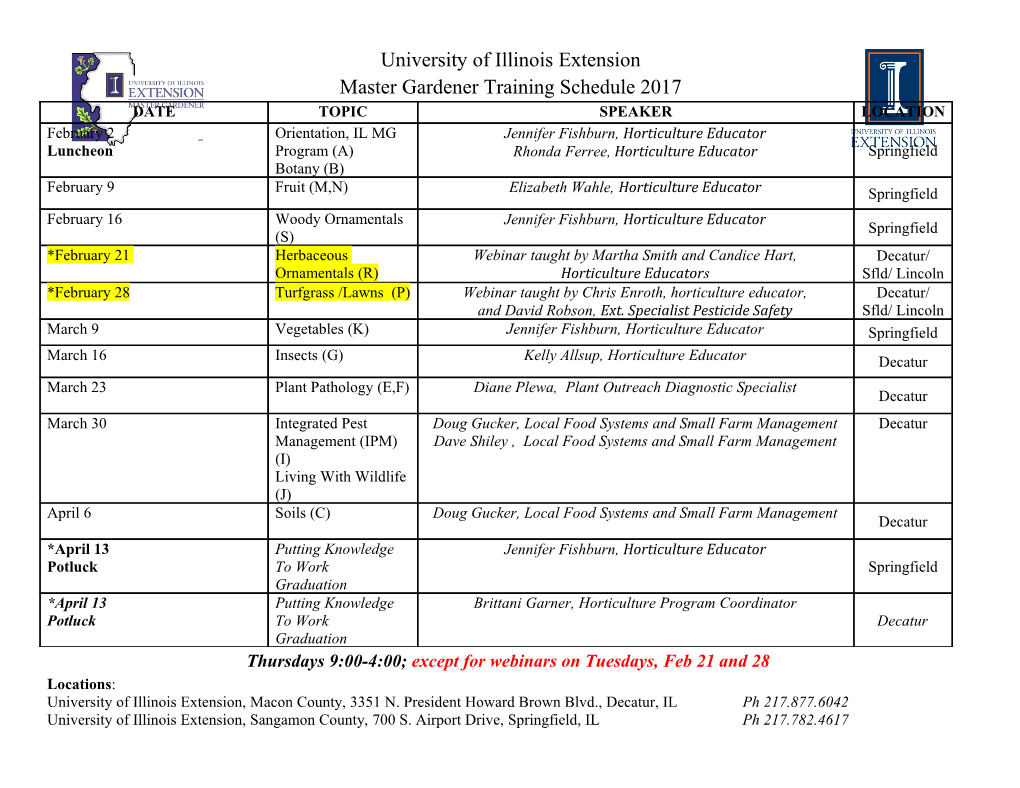
Page 1 of 42 Molecular Ecology Resources 1 Simultaneous identification of host, ectoparasite and 2 pathogen DNA via in-solution capture 3 4 Michael G. Campana* ,1 , Melissa T. R. Hawkins* ,1,2 , Lauren H. Henson 1, Kristin 5 Stewardson 1, Hillary S. Young 3, Leah R. Card 4, Justin Lock 1, Bernard 6 Agwanda 5, Jory Brinkerhoff 6, Holly D. Gaff 7, Kristofer M. Helgen 2, Jesús E. 1, 2 4 1,§ 7 Maldonado , William J. McShea , Robert C. Fleischer 8 *Co-first Authors; §Corresponding author: [email protected] 9 1Center for Conservation and Evolutionary Genetics, Smithsonian Conservation Biology Institute, 10 3001 Connecticut Avenue NW, Washington, DC 20008, USA 11 2Division of Mammals, National Museum of Natural History, MRC 108, Smithsonian Institution, P.O. 12 Box 37012, Washington, DC 20013-7012, USA 13 3Department of Ecology, Evolution and Marine Biology, University of California Santa Barbara, Santa 14 Barbara, CA 93106, USA 15 4Smithsonian Conservation Biology Institute, National Zoological Park, 1500 Remount Rd., Front 16 Royal, VA 22630, USA 17 5National Museums of Kenya, Box 40658-00100, Nairobi, Kenya 18 6Department of Biology, University of Richmond, B322 Gottwald Center for the Sciences, 28 19 Westhampton Way, University of Richmond, VA 23173, USA 20 7Department of Biological Sciences, Old Dominion University, Norfolk, VA 23529, USA 21 22 Keywords: Ectoparasite, blood meal, pathogen, in-solution capture, DNA, high-throughput 23 sequencing 24 Running Title: Host, ectoparasite and pathogen capture 1 Molecular Ecology Resources Page 2 of 42 25 Abstract 26 Ectoparasites frequently vector pathogens from often unknown pathogen reservoirs to 27 both human and animal populations. Simultaneous identification of the ectoparasite species, 28 the wildlife host that provided their most recent blood meal(s), and their pathogen load would 29 greatly facilitate the understanding of the complex transmission dynamics of vector-borne 30 diseases. Currently, these identifications are principally performed using multiple polymerase 31 chain reaction (PCR) assays. We developed an assay (EctoBaits) based on in-solution capture 32 paired with high-throughput sequencing to simultaneously identify ectoparasites, host blood 33 meals, and pathogens. We validated our in-solution capture results using double-blind PCR 34 assays, morphology, and collection data. The EctoBaits assay effectively and efficiently 35 identifies ectoparasites, blood meals, and pathogens in a single capture experiment, allowing 36 for high-resolution taxonomic identification while preserving the DNA sample for future 37 analyses. 38 39 Introduction 40 Nearly 30% of emerging infectious diseases of humans are vector-borne, with most of 41 these having a wildlife reservoir host (Jones et al . 2008). Ectoparasites are common vectors of 42 pathogen transmission from one host to another, involved in diseases such as Lyme disease 43 (Borrelia burgdorferi ), Rocky Mountain spotted fever (Rickettsia rickettsii), malaria 44 (Plasmodium spp.) and anaplasmosis ( Anaplasma phagocytophilum ), among many others 45 (e.g. Pfäffle et al. 2013; Brites-Neto et al. 2015). Dynamics of disease transmissions are 46 changing at an unprecedented rate due to anthropogenic effects on ecosystems including 47 modification of landscapes, alteration of species distributions, and impacts of human- 48 mediated climate change (e.g. Jones et al. 2008; Hoberg & Brooks 2015). In order to 2 Page 3 of 42 Molecular Ecology Resources 49 understand disease dynamics and the prevalence of specific diseases, intensive field studies 50 are required to collect and screen host and ectoparasite samples for a variety of pathogens 51 (Gilbert & Jones 2000; Lynen et al. 2007; Midilli et al. 2009; Sabatini & Pinter 2010). By 52 monitoring the prevalence and distribution of pathogens across hosts, ectoparasites, and 53 habitats, we can better predict disease spread, improve our understanding of cycles of disease 54 transmission, and better document movement of pathogens through landscapes, with 55 important applications for predicting emergence of zoonoses globally. 56 Researchers exploring the dynamics of vector-borne disease in the field often face 57 multiple challenges in correctly identifying hosts, ectoparasites, and pathogens (Allan et al. 58 2010). Precise morphological identification of hosts and ectoparasites often requires 59 significant taxonomic expertise (e.g. Nadolny et al . 2011; Young et al . 2015a), while 60 molecular techniques are required for accurate and reliable screening of pathogens (Pichon et 61 al. 2003; Nadolny et al. 2011, 2014). Damage to field-collected specimens can complicate 62 taxonomic identification. Furthermore, ectoparasite samples often yield small volumes and 63 low concentrations of extracted DNA (laboratory observations, Allender et al. 2004). 64 Multiple polymerase chain reaction (PCR) assays are thus frequently needed to ensure 65 accurate host and pathogen identification, which can rapidly consume these small quantities 66 of DNA. While multiplex PCR assays can reduce the total number of reactions (e.g. Courtney 67 et al . 2004), these strategies require extensive optimization and the use of expensive reagents 68 (e.g. fluorescent probes) or additional experimental steps (e.g. bacterial cloning). Therefore, 69 efficient molecular screening methods are needed to identify DNAs corresponding to a 70 comprehensive number of potential pathogens as well as a variety of hosts and parasites. 71 DNA capture assays coupled with next generation sequencing are highly suitable for 72 the study of pathogens from a variety of low copy number sources including ectoparasite, 3 Molecular Ecology Resources Page 4 of 42 73 non-invasive, museum and ancient samples (Bos et al. 2014, and references therein; Hawkins 74 et al . 2015; Vuong et al . 2013). We developed a method to simultaneously enrich samples 75 from various sources for ectoparasite (primarily ixodid ticks), blood meal, and pathogen 76 DNA. Here we describe an in-solution capture assay (EctoBaits) that, paired with high- 77 throughput sequencing, can simultaneously identify a range of ectoparasites, hosts, and 78 pathogen strains from a single library preparation and in-solution hybridization experiment. 79 The EctoBaits assay capitalizes on the wealth of data provided in current DNA barcoding 80 databases to provide accurate species-level taxonomic assignment. While currently limited to 81 northeastern American and East African taxa, the EctoBaits assay can be easily adapted and 82 expanded for other regions of interest. 83 84 Materials and Methods 85 Probe Design 86 With the goal of designing an in-solution capture assay for detecting ectoparasites, 87 blood meals, and pathogens simultaneously, we comprehensively searched public databases 88 (BOLD and Genbank) for sequences from a variety of taxa relevant to ongoing studies in the 89 northeastern United States and East Africa (Table S1), where we are engaged in ongoing 90 fieldwork (Card et al ., in revision; Young et al . 2014, 2015a). Both regions are hotspots of 91 vector-borne disease risk (Jones et al . 2008). For ectoparasites, hosts, macroparasites, and 92 eukaryotic pathogens, we targeted mitochondrial genes due to their higher copy number per 93 cell and corresponding increased likelihood of successful enrichment compared to nuclear 94 genes. For bacterial pathogens, we targeted diagnostic regions (such as 16S rRNA, citrate 95 synthase, flagellin and IS1111 spacers) that had been identified as species-specific in previous 96 research (Bai et al . 2011, Eremeeva et al . 1994, Ghafar & Eltablawy 2011, Levin & Fish 4 Page 5 of 42 Molecular Ecology Resources 97 2000, Mediannikov et al . 2010). The final bait targets included 111 American bird species, 47 98 American mammal species, 108 African mammal species, 84 pathogen strains (59 bacterial 99 strains, 25 eukaryotic strains), 53 hard tick (Ixodidae) species, one soft tick (Argasidae) 100 species, 10 flea species, one sucking louse (Anoplura) species, and one filiarial parasite. 101 Pathogen baits were derived from eight Anaplasma strains, five Babesia strains, seven 102 Bartonella strains, three Borrelia strains, two Coxiella strains, 21 Ehrlichia strains, 11 103 Hepatozoon strains, 17 Rickettsia strains, nine Theileria strains, and one strain of Yersinia 104 pestis (Table S1) . 105 To remove overly similar redundant sequences (<10% divergence) from the probe set, 106 the identified sequences were clustered using CD-HIT-EST (Li & Godzik 2006). Clustering 107 the sequences produced a degenerate set designed to capture a wide range of species. The 108 clustered sequences were split into 100 bp pieces for probe synthesis, resulting in 3,901 109 different probe sequences after quality control (Supporting Information). Probes did not 110 overlap and were not tiled. Each probe sequence was copied approximately five times to fill a 111 20,000-probe MYbaits kit (MYcroarray, USA). The effectiveness of the probe set was 112 confirmed by spiking certified pathogen-free chicken ( Gallus gallus ) and rabbit ( Oryctolagus 113 cuniculus ) blood with known concentrations of pathogen-specific PCR products and 114 capturing these samples using EctoBaits (see Supporting Information). 115 116 Field Collection and Sample Selection 117 Specimens were obtained from large ongoing investigations of ectoparasites and 118 vector-borne diseases in dry tropical savanna ecosystem of Laikipia County, Kenya (0°17′N, 119 36°52′ E) and forest and suburban ecosystems
Details
-
File Typepdf
-
Upload Time-
-
Content LanguagesEnglish
-
Upload UserAnonymous/Not logged-in
-
File Pages42 Page
-
File Size-