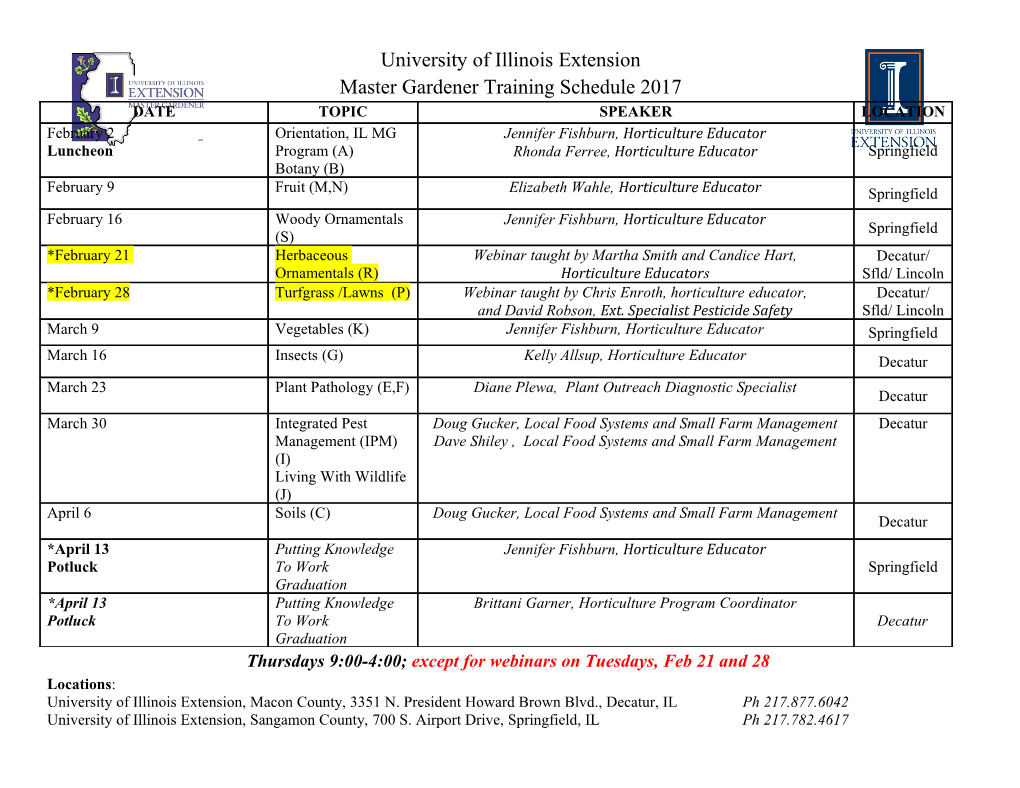
manuscript submitted to JGR: Atmospheres 1 Aircraft Charging and its Influence on Triggered 2 Lightning 1 2 2 1 1 3 C. Pavan , P. Fontanes , M. Urbani , N. C. Nguyen , M. Martinez-Sanchez , 1 2 1 4 J. Peraire , J. Montanya , and C. Guerra-Garcia 1 5 Department of Aeronautics and Astronautics, Massachusetts Institute of Technology, Cambridge, 6 Massachusetts. 2 7 Department of Electrical Engineering, Universitat Polit`ecnicade Catalunya, Terrassa (Barcelona), Spain. 8 Key Points: 9 • Aircraft triggered lightning is influenced by the net charge of the floating body 10 • Positive leader inception from an aircraft requires higher amplitude external fields 11 when negatively biasing the vehicle 12 • Experimental results validate a proposed method of aircraft triggered lightning 13 strike risk-reduction Corresponding author: C. Guerra-Garcia, [email protected] {1{ manuscript submitted to JGR: Atmospheres 14 Abstract 15 This paper reports on a laboratory experiment to study the effect of vehicle net charge 16 on the inception of a positive leader from an aircraft exposed to high atmospheric elec- 17 tric fields. The experiment models the first stage of aircraft-triggered lightning, in which 18 a positive leader typically develops from the vehicle and is shortly afterwards followed 19 by a negative leader. This mechanism of lightning initiation amounts to around 90% of 20 strikes to aircraft. Aircraft can acquire net charge levels of the order of a millicoulomb 21 from a number of sources including corona emission, charged particles in the engine ex- 22 haust and charge transfer by collisions with particles in the atmosphere. In addition, air- 23 craft could potentially be artificially charged through controlled charge emission from 24 the surface. Experiments were performed on a model aircraft with a 1m wingspan, which 25 was suspended between two parallel electrodes in a 1.45m gap with voltage difference 26 of a few hundred kilovolts applied across it. In this configuration, it is found that the 27 breakdown field can vary by as much as 30% for the range of charging levels tested. The 28 experimental results show agreement with an electrostatic model of leader initiation from 29 aircraft, and the model indicates that the effect can be substantially stronger if additional 30 negative charge is added to the aircraft. The results from this work suggest that flying 31 uncharged is not optimal in terms of lightning avoidance and open up the possibility of 32 developing risk-reduction strategies based on net charge control. 33 Plain Language Summary 34 Commercial aircraft are typically struck by lightning around once per year, and the 35 vast majority of these events are triggered by the aircraft itself. The lightning discharge 36 originates on the surface of the aircraft in areas with sharp edges. Whether a discharge 37 develops is in part due to the net electric charge of the aircraft, which can be acquired 38 both naturally or artificially. Previous work has shown that it is theoretically possible 39 to reduce the likelihood of a lightning strike occurring by manipulating the net charge 40 of the aircraft. In this paper, the authors perform laboratory experiments to validate this 41 hypothesis. These experiments demonstrate that the threshold for lightning could be in- 42 creased by 30% by charging the aircraft negatively, which means that an aircraft could 43 fly safely through ambient electric fields that are around 30% higher than those of an 44 uncharged baseline. Theoretical estimates suggest that further improvement may be pos- 45 sible if the aircraft were charged to a more negative state than those tested. This work 46 gives laboratory scale experimental evidence that it is possible to reduce the frequency 47 of lightning strikes on aircraft by manipulating their charge, and encourages further in- 48 vestigation of the proposed lightning strike risk-reduction strategy. 49 1 Introduction 50 Formal studies into lightning effects and aircraft survivability began in the 1940s 51 after the catastrophic accident of a Pennsylvania-Central Airlines DC-3A aircraft (Plumer, 52 2017). Nowadays, strict protection and mitigation measures are embedded in aircraft: 53 the use of expanded metal foil or wire mesh to ensure a fully conductive path and elec- 54 tromagnetic shielding in composite structures, incorporation of lightning diverter strips 55 in the radome, securing of fasteners and joints to avoid arcing and sparking in the fuel 56 tanks, wire bundle shielding, proper grounding, and the use of surge protectors (Sweers 57 et al., 2012). These measures ensure the safety of flight in the event of a strike and must 58 comply with regulations of the civil certification authorities (the Federal Aviation Ad- 59 ministration, FAA, and the European Aviation Safety Agency, EASA). Standard com- 60 mittees (the Society of Automotive Engineers, SAE, and the European Organization for 61 Civil Aviation Equipment, EUROCAE) provide detailed guidelines on testing standards 62 to demonstrate compliance (section 2.1). Although lightning strikes pose no critical safety 63 concerns, they are responsible for costly delays, service interruptions, and repairs. {2{ manuscript submitted to JGR: Atmospheres (1) (2) + + E ∞ E − − (3) (4) E ¡ − −− − Figure 1. Bidirectional uncharged leader formation from an aircraft exposed to atmospheric electric fields. See the 4th paragraph of section 1 for detailed discussion. 64 It is generally quoted that, on average, commercial aircraft are struck by lightning 65 about once per year, a figure that is supported by both instrumented flight campaigns 66 and commercial operations (F. A. Fisher & Plumer, 1977). The frequency of lightning 67 strikes that a particular aircraft experiences will of course depend on factors such as its 68 flight envelope (geographic area of operation, cruise altitude, duration of climb and de- 69 scent) and size. 70 From an operational perspective, the highest probabilities for lightning strikes have 71 been observed during climb and descent at an altitude of 5000-15000 feet. Most light- 72 ning strikes to airplanes occur close to freezing temperatures and during precipitation 73 but not necessarily in the presence of an active thunderstorm (Rakov & Uman, 2003). 74 From an electrostatic perspective, lightning initiation from aircraft, or aircraft-triggered 75 lightning (Moreau et al., 1992), can be explained using the bidirectional uncharged leader 76 concept (Kasemir, 1950; Mazur, 1988, 1989). This mechanism, responsible for about 90% 77 of strikes, was verified using airborne data from the NASA F-106B (B. D. Fisher & Plumer, 78 1984) and FAA CV-580 (Reazer et al., 1987) campaigns. The sequence of events that 79 precede a lightning strike is shown schematically in figure 1: (1) the aircraft is polarized 80 when exposed to atmospheric electric fields, resulting in the amplification of the local 81 electric fields at its extremities; (2) a positive leader is triggered due to the lower incep- 82 tion and propagation thresholds for the positive polarity; (3) charge conservation forces 83 the aircraft to more negative charge levels, further enhancing the local electric fields at 84 the negatively charged extremities; (4) finally the threshold for negative leader incep- 85 tion and propagation is reached and a negative leader follows. If the leaders connect with 86 others originating from clouds or the ground, a damaging high current arc forms. Viewed 87 from this electrostatic perspective (Lalande et al., 1999b), to first order, the probabil- 88 ity of aircraft-triggered lightning will only depend on the geometry of the vehicle, the 89 external fields, the atmospheric conditions (which will modify the inception thresholds), 90 and the net vehicle charge. 91 In this work the influence of the vehicle's net charge on leader inception is exper- 92 imentally demonstrated, confirming recent theoretical estimates by Guerra-Garcia et al. 93 (2017, 2018). These results are relevant since aircraft can acquire net charge from a num- {3{ manuscript submitted to JGR: Atmospheres 94 ber of sources including friction with the runway during takeoff, corona discharges from 95 static dischargers or other electrically stressed regions, charged species in the engine ex- 96 haust, and charge transfer by collision with particles in the atmosphere (Vonnegut & Lit- 97 tle, 1965). In addition, the results from this work may open up a pathway towards an 98 active means of reducing the risk of aircraft-triggered lightning through charge control 99 (Martinez-Sanchez et al., 2019). 100 The paper is organized as follows. It begins with an overview of aircraft lightning 101 leader attachment testing standards, followed by a description of the modifications in- 102 corporated to allow for the exploration of the effect of the vehicle's net charge. Next, ex- 103 perimental results of the dependence of lightning inception on aircraft charge are pre- 104 sented and compared to a theoretical model. The paper closes with a discussion on how 105 the electrostatic perspective of aircraft-triggered lightning can assist the development of 106 lightning detection and prediction, and ultimately, risk-reduction technologies. 107 2 Testing Procedures 108 2.1 Overview of Lightning Leader Attachment Testing 109 Aircraft components must undergo a variety of tests for susceptibility to environ- 110 mental damage. In particular, testing standards representative of flight conditions lead- 111 ing up to and during a strike are described in the SAE standard ARP5416 (SAE Aerospace, 112 2005) and the equivalent European standard EUROCAE ED-105 (EUROCAE, 2005). 113 The impact of strikes to aircraft are typically classified as either direct effects, encom- 114 passing any risks associated with the lightning discharge itself; or indirect effects, which 115 arise from interactions between the electromagnetic fields generated by the discharge and 116 components of the aircraft. Direct effects mostly correspond to physical damage to the 117 external surfaces of the aircraft, while indirect effects include damage to sensors and elec- 118 tronics (Uman, 2008; Plumer, 2012).
Details
-
File Typepdf
-
Upload Time-
-
Content LanguagesEnglish
-
Upload UserAnonymous/Not logged-in
-
File Pages21 Page
-
File Size-