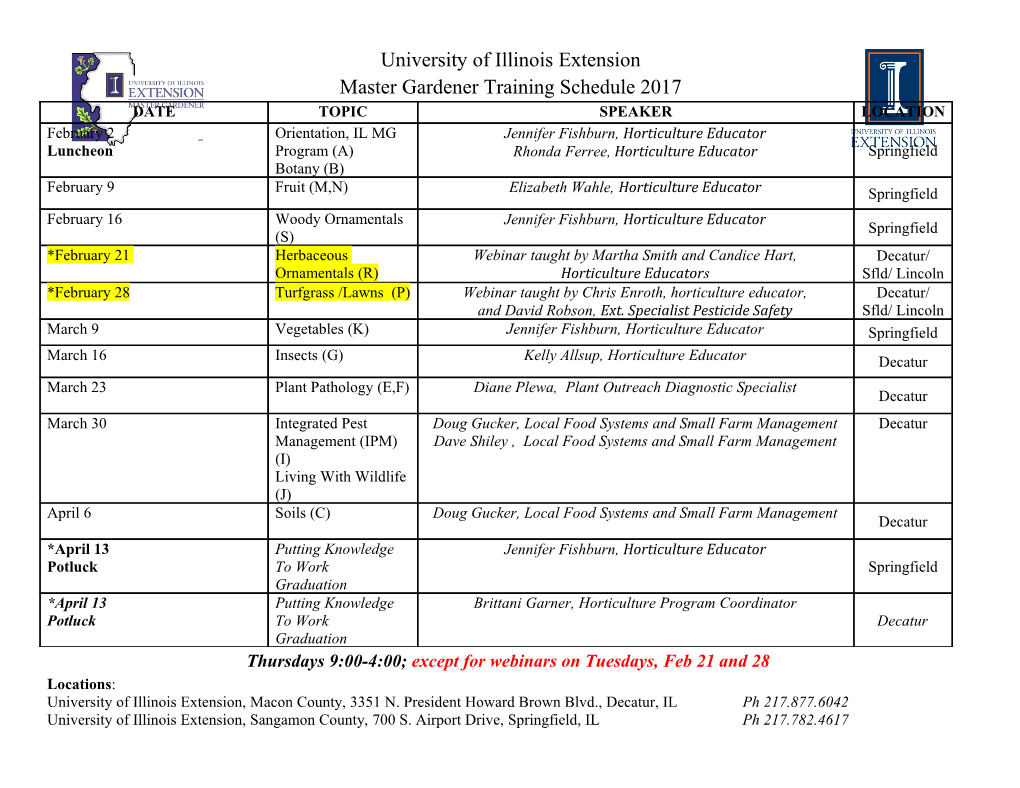
METHIONINE SULFOXIDE REDUCTASE DEFICIENCY LEADS TO MITOCHONDRIAL DYSFUNCTION IN DROSOPHILA MELANOGASTER by Jennifer Verriotto A Thesis Submitted to the Faculty of The Charles E. Schmidt College of Science in Partial Fulfillment of the Requirements for the Degree of Master of Science Florida Atlantic University Boca Raton, Florida March 2011 METHIONINE SULFOXIDE REDUCTASE DEFICIENCY LEADS TO MITOCHONDRIAL DYSFUNCTION IN DROSOPHILA MELANOGASTER by Jennifer Verriotto This thesis was prepared under the direction of the candidate's thesis advisor, Dr. David Binninger, Department of Biological Sciences, and has been approved by the members of her supervisory committee. It was submitted to the faculty of the Charles E. Schmidt College of Science and was accepted in partial fulfillment of the requirements for the degree of Master of Science. SUPERVIS Gary W. Pe ,Ph.D. Dean, The Charles E. chmidt allege of Science £~r%-/7?~ ii ACKNOWLEDGEMENTS I would like to thank my advisor Dr. David Binninger for his guidance and constant confidence in my work and me. I would also like to thank everyone in the lab who helped me along the way. iv ABSTRACT Author: Jennifer Verriotto Title: Methionine Sulfoxide Reductase Deficiency Leads to Mitochondrial Dysfunction in Drosophila melanogaster Institution: Florida Atlantic University Thesis Advisor: Dr. David Binninger Degree: Master of Science Year: 2011 Mitochondria are a major source of reactive oxygen species and are particularly vulnerable to oxidative stress. Mitochondrial dysfunction, methionine oxidation, and oxidative stress are thought to play a role in both the aging process and several neurodegenerative diseases. Two major classes of methionine sulfoxide reductases, designated MsrA and MsrB are enzymes that function to repair the enatiomers of methionine sulfoxide, met-(o)-S and met-(o)- R, respectively. This study focuses on the effect of Msr deficiencies on mitochondrial function by utilizing mutant alleles of MsrA and MsrB. The data show that loss of only one form of Msr in the mitochondria does not completely impair the function of the mitochondria. However, loss of both Msr proteins within the mitochondria leads to an increased ROS production and a diminished energy output of the mitochondria. These results support the hypothesis that Msr plays a key role in proper mitochondrial function. v METHIONINE SULFOXIDE REDUCTASE DEFICIENCY LEADS TO MITOCHONDRIAL DYSFUNCTION IN DROSOPHILA MELANOGASTER List of Tables ........................................................................................................ vii List of Figures ...................................................................................................... viii Chapter One – Background and Introduction ........................................................ 1 Aging and Oxidative Damage .................................................................... 1 Oxidative Damage and Mitochondrial Function .......................................... 2 Mitochondria and Neurodegenerative Diseases ........................................ 4 Methionine Sulfoxide Reductases (Msrs) History, Function and Characteristics ............................................................................................ 5 The Msr System and the Mitochondria ....................................................... 7 Binninger Lab Studies MsrA and MsrB in Drosophila ......................... …..10 Chapter Two – Materials and Methods……………………………………………...12 Msr Deficient Drosophila melanogaster Lines…………………………...…12 Creation of MsrB Deficiency Cross ................................................ 12 Creation of Control and Msr Null Mutants ..................................... 13 Creation of MsrA Deficiency Cross ............................................... 14 Molecular and Biochemical Characterization ........................................... 15 Protein Isolation ……………………………………………………….16 vi Mitochondrial Isolation .................................................................. 16 SDS- PAGE .................................................................................. 16 Mitochondrial ATP Synthesis ....................................................... 17 Mitochondrial ROS Production ..................................................... 18 Phenotypic Characterization ..................................................................... 18 Climbing Assay .............................................................................. 18 Chapter Three – Results ..................................................................................... 20 Protein Expression Results ........................................................... 22 Climbing Assay Results ................................................................. 23 Mitochondrial ATP Production Results .......................................... 24 Mitochondrial Expression Results ................................................. 27 Mitochondrial ROS Production Results ......................................... 28 Chapter Four- Discussion .................................................................................... 29 Creation of Mutant Lines .......................................................................... 30 ATP and ROS Production ......................................................................... 31 Negative Geotaxis Response ................................................................... 32 Summary and Future Studies ................................................................... 33 Reference………………………………………………………………………………35 vi TABLES Table 1. Genotype of Stocks ........................................................................... 14 Table 2. Percentage Difference Between Mutant Strains and Control………...29 vii FIGURES Figure 1. Creation of the MsrB deficient cross ................................................. 13 Figure 2. Morphology of Msr-deficient animals and control .............................. 21 Figure 3. Western blot analysis ........................................................................ 22 Figure 4. Locomotor assay…………………………………………………………23 Figure 5. ATP production ................................................................................ 25 Figure 6. ATP synthase .................................................................................... 26 Figure 7. Mitochondrial Western blot analysis .................................................. 27 Figure 8. ROS-production in flies lacking Msr activity ...................................... 28 viii I. BACKGROUND AND INTRODUCTION Aging and Oxidative Damage. Aging is a time-dependent phenomenon characterized by a decline in physiological function among different organisms. Several theories have been proposed to explain the aging process. One of the most important theories is known as the free radical theory [1, 2]. It hypothesizes that free radicals produced during aerobic respiration cause oxidative damage to macromolecules (e.g., proteins, nucleic acids, lipids) resulting in aging and ultimately death. Considerable evidence suggests that oxidative damage might be related to neurodegenerative diseases and might contribute to a shortened lifespan [3- 5]. This damage may occur from imbalances between oxidant production and repair processes in the different cellular compartments of eukaryotic cells, especially in mitochondria [6]. Mitochondria consume up to 90% of cellular oxygen to support oxidative phosphorylation and synthesis of ATP. Only 1 -2% of the oxygen consumed by the mitochondrial respiratory chain leads to oxygen radical production and hydrogen peroxide formation [7]. This reactive oxygen species (ROS) production could induce a change in cellular function by damaging the cellular macromolecules that accumulate during aging and neurodegenerative diseases progression. 1 ROS Production in Mitochondria. The generation of mitochondrial ROS (including superoxide anion radical (O2 ·), hydroxyl radical (·OH), and hydrogen peroxide H2O2)) is the result of oxidative phosphorylation in the electron transport chain, a process that uses the controlled oxidation of NADH or FADH to generate a potential energy for protons across the mitochondrial inner membrane through complexes I, III, and IV. This potential energy is then coupled to ATP synthesis by complex V. During this process, oxygen normally serves as the ultimate electron acceptor and is reduced to water. However, electrons that leak to oxygen through complexes I and III could generate superoxide anion for ROS production [8]. There is considerable experimental evidence that the two major sites for ROS production are complexes I and III where large changes between the potential energy of the electrons and oxygen reduction take place [9,10]. Consequently, mitochondria are a major source of cellular ROS production and a target for oxidative damage. ROS and Mitochondrial Function. In aging and disease conditions, cellular ROS levels increase, leading to accumulation of oxidative damage to the mitochondria, impaired calcium buffering, and decrease of ATP production. ATP is indispensable for the body because it maintains chemical gradients by driving channels such as ion pumps, reuptake of neurotransmitters from the synaptic cleft between neurons, as well as acting as the driving force of a multitude of cellular processes. When ATP declines to harmful levels, a massive outflow of excitatory neurotransmitters occurs leading to an increase of ROS [11, 12]. In 2 addition to ATP synthesis, mitochondria are the
Details
-
File Typepdf
-
Upload Time-
-
Content LanguagesEnglish
-
Upload UserAnonymous/Not logged-in
-
File Pages56 Page
-
File Size-