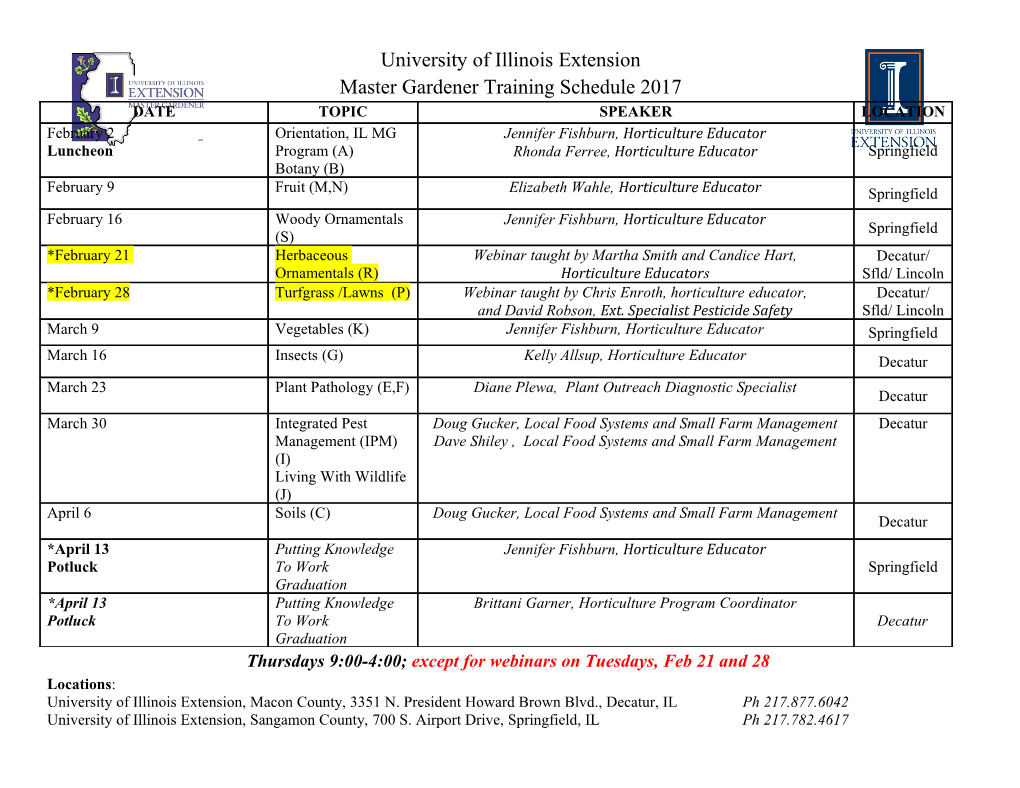
Physiologic Effects of Noninvasive Ventilation Neil R MacIntyre Introduction NIV Can Augment Minute Ventilation NIV Unloads Ventilatory Muscles NIV Resets the Ventilatory Control System Alveolar Recruitment and Gas Exchange Other Physiologic Effects of NIV: Intended and Unintended Maintaining Upper-Airway Patency Reducing Imposed Triggering Loads From Auto-PEEP Cardiac Interactions: Both Beneficial and Harmful Ventilator-Induced Lung Injury Production of Auto-PEEP Patient-Ventilator Interactions Summary Noninvasive ventilation (NIV) has a number of physiologic effects similar to invasive ventilation. The major effects are to augment minute ventilation and reduce muscle loading. These effects, in turn, can have profound effects on the patient’s ventilator control system, both acutely and chron- ically. Because NIV can be supplied with PEEP, the maintenance of alveolar recruitment is also made possible and the triggering load imposed by auto-PEEP can be reduced. NIV (or simply mask CPAP) can maintain upper-airway patency during sleep in patients with obstructive sleep apnea. NIV can have multiple effects on cardiac function. By reducing venous return, it can help in patients with heart failure or fluid overload, but it can compromise cardiac output in others. NIV can also increase right ventricular afterload or function to reduce left ventricular afterload. Potential det- rimental physiologic effects of NIV are ventilator-induced lung injury, auto-PEEP development, and discomfort/muscle overload from poor patient–ventilator interactions. Key words: invasive ventilation; noninvasive ventilation; minute and alveolar ventilation; ventilation distribution; ventilation-perfusion match- ing; control of ventilation; ventilatory muscles; work of breathing; patient–ventilator interactions; ventilator- induced lung injury. [Respir Care 2019;64(6):617–628. © 2019 Daedalus Enterprises] Introduction this definition, this discussion will focus on positive-pres- sure devices with a mask interface to either totally or partially Noninvasive ventilation (NIV) is the process of support- ing respiration using devices that do not require an artifi- cial airway. While a variety of external chest devices meet Dr MacIntyre presented a version of this paper at the 57th RESPIRATORY CARE Journal Conference, held June 14-15, 2018, in St Petersburg, Florida. Dr MacIntyre is affiliated with the Division of Pulmonary and Critical Care Correspondence: Neil R MacIntyre MD FAARC, Division of Pulmonary Medicine, Duke University Medical Center, Durham, North Carolina. and Critical Care Medicine, Duke University Medical Center, Box 3911, Durham, NC 27710. E-mail: [email protected]. Dr MacIntyre has disclosed relationships with Ventec, Breathe, and InspiRx. DOI: 10.4187/respcare.06635 RESPIRATORY CARE • JUNE 2019 VOL 64 NO 6 617 PHYSIOLOGIC EFFECTS OF NIV ˙ provide O2 and CO2 transport between the environment and ther way, the minute ventilation (VE) delivered to the pa- the pulmonary capillary bed.1,2 NIV is often coupled with tient can be increased substantially. ˙ PEEP to maintain positive airway pressure throughout the Unless there is a marked increase in dead space (VD) ˙ ventilatory cycle. The primary desired effect of NIV is to with positive-pressure ventilation, an increased VE from maintain adequate levels of PO and PCO in arterial blood NIV usually translates to an increased alveolar ventilation 2 2 ˙ ϭ ˙ Ϫ ˙ ˙ while also unloading the inspiratory muscles. (VA VE VD). The net effect of an increased VA is to NIV has many of the same physiologic effects as inva- provide additional O2 to and remove additional CO2 from ˙ sive ventilation (ie, through an artificial airway). However, alveolar gas. Mathematically, the relationship of VA to there are important differences. First, because of inherent alveolar gas partial pressures are: leaks, NIV systems cannot always supply volumes and pressures comparable to invasive ventilation, despite so- PaO ϭ PIO Ϫ ͑V˙ O /V˙ A͒ ϫ k phisticated leak-compensation features. These leaks can 2 2 2 also affect triggering sensitivity and patient–ventilator syn- P ϭ ͑V˙ /V˙ ͒ ϫ k chrony during flow delivery and breath cycling. Second, aCO2 CO2 A NIV is applied to the oronasal pharynx that connects to the esophagus as well as the trachea. Despite the presence of where P is inspired oxygen, V˙ is total body oxygen IO2 O2 gastroesophageal sphincters, high positive esophageal pres- consumption, V˙ is total body CO production, and k is CO2 2 sures can lead to gastric distention. Third, the unprotected a constant. These relationships are depicted in Figure 1 trachea, especially in the setting of a distended stomach, is and show that, as V˙ increases, alveolar P asymptotes on A O2 exposed to significant aspiration risk. Fourth, the absence the P and alveolar P asymptotes on zero.10 IO2 CO2 of an artificial airway also limits the effectiveness of air- Gas transport across the alveolar capillary membrane is way suctioning and pulmonary toilet. Fifth, with single- driven by gradients between alveolar and venous blood lumen circuits relying on controlled leaks for exhalation, gas tensions and alveolar capillary membrane diffusing the potential for CO2 re-breathing exists, especially if flow properties. In general, with a normal blood transit time in settings are low. Finally, inspiratory pressure settings on the capillary bed of Ͻ 1 s, gas diffusion is sufficiently many dedicated NIV systems are referenced to atmosphere, rapid and equilibration of both CO2 and O2 in the alveolus which can lead to confusion in clinicians more familiar and capillary bed is complete.11-12 with invasive ventilation devices where inspiratory pres- The ultimate PO and PCO in pulmonary venous blood 2 2 ˙ sure is referenced to set expiratory pressure (PEEP). entering the left atrium, however, depends not only on VA There are also important advantages to NIV vs invasive and alveolar-capillary gas transport, but also on ventilation ventilation. By using a noninvasive mask interface instead perfusion matching (V˙ /Q˙ ) relationships throughout the mil- ˙ 11-13 of an artificial translaryngeal airway, preservation of glot- lions of lung units into which the VE distributes. Factors tic function may, in fact, reduce aspiration risk from pha- affecting this distribution include regional resistances, com- ryngeal material. Intermittent breaks from NIV are possi- pliances, functional residual capacities, and the machine-de- ble, which allows talking and swallowing, and this, coupled livered pressure/flow pattern (square vs decelerating vs vari- with the absence of an irritating trans-laryngeal airway, may able flow; with or without an inspiratory pause; with or without improve comfort and reduce sedation needs compared to in- expiratory pressure). In general, positive-pressure breaths will vasive ventilation.3 NIV can also be helpful in maintaining tend to distribute more to units with high compliance and low patency of upper-airway obstruction in patients with obstruc- resistance and away from obstructed or stiff units. This cre- tive sleep apnea and can reduce venous return and pulmonary ates the potential for regional overdistention of healthier lung edema in selected patients with congestive heart failure.4,5 units in heterogeneous disease states, even in the face of Importantly, like invasive ventilation, NIV can also injure the normal tidal volumes (see ventilator-induced lung injury dis- 6-8 ˙ lungs if used inappropriately. The specific physiologic ef- cussion below). VE distribution is also affected by body po- fects of NIV on both the respiratory system as well as im- sition (eg, gravitational forces tend to distend non-dependent portant non-respiratory systems (ie, neurologic and cardio- regions) and the presence or absence of inspiratory muscle vascular) are reviewed below. activity (eg, an actively contracting diaphragm tends to dis- tribute gas to dependent regions better).14-15 V˙ /Q˙ effects are different for CO and O .11,12 Because NIV Can Augment Minute Ventilation 2 2 CO2 is very soluble in blood and CO2 content is essentially linearly related to P , the ultimate P in pulmonary ve- CO2 CO2 By adding pressure and flow to the mask interface using nous blood is a flow-weighted average of all lung units in NIV, tidal volumes can be either created during a con- which alveolar gas came into at least some contact with cap- trolled (ie, machine time-triggered) breath or augmented illary blood (ie, V˙ ). On the other hand, the ultimate P in A O2 during assisted (ie, patient effort-triggered) breaths.9 Ei- pulmonary venous blood is not a flow-weighted average but 618 RESPIRATORY CARE • JUNE 2019 VOL 64 NO 6 PHYSIOLOGIC EFFECTS OF NIV Arterial pH 7.7 PaCO2 7.6 Alveolar PO2 SaO 7.5 2 Alveolar PCO2 7.4 7.3 7.2 7.1 130 120 (mm Hg) Arterial pH 2 CO 110 or P 2 O 100 Alveolar P 90 80 70 60 content (volume %) 2 50 40 Arterial CO 30 (%) 2 20 aO S 10 0 1 234 567891011 Alveolar ventilation (L/min) Fig. 1. Relationship of alveolar ventilation to alveolar P and P and to the ultimate S and P with an assumed oxygen consumption O2 CO2 aO2 aCO2 of 250 mL/min and CO2 production of 200 mL/min. The shaded area represents a normal alveolar ventilation. Note that as alveolar ventilation increases, alveolar P decreases exponentially, and P follows. In contrast, as alveolar ventilation increases, the alveolar CO2 aCO2 P increases and approaches the inspired P . However, because hemoglobin saturation is virtually complete at P values Ͼ 70– O2 O2 O2 80 mm Hg, arterial oxygen content
Details
-
File Typepdf
-
Upload Time-
-
Content LanguagesEnglish
-
Upload UserAnonymous/Not logged-in
-
File Pages12 Page
-
File Size-