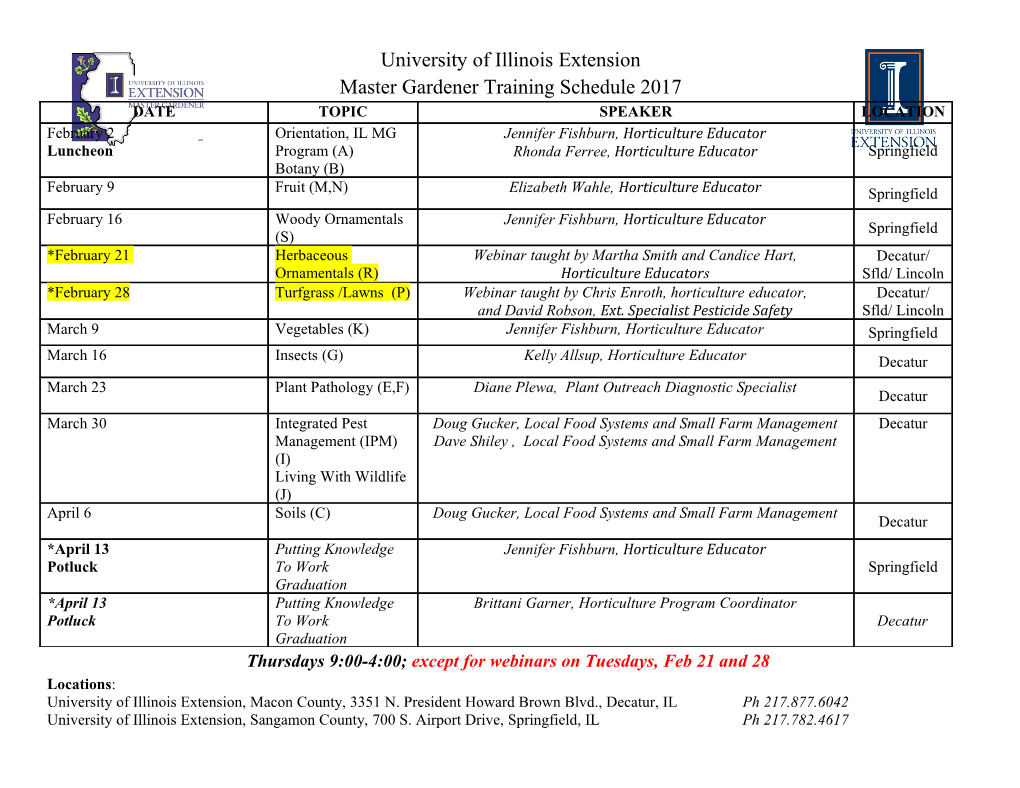
H.-J. Freund, U. Biittner, B. Cohen and J. Noth Progress in Brain Research, Vol. 64. 0 1986 Elsevier Science Publishers B.V. (Biomedical Division) Spinal programs for locomotion Gerald E. Loeb Laboratory of Neural Control, IRP, National Institute of Neurological and Communicative Disorders and Stroke, National Institutes of Health, 9000 Rockville Pike, Bethesda, MD 20205, U.S.A. Introduction is attached to an initially stationary but movable object, then the object moves when the muscle con- In comparing the current level of knowledge re- tracts, providing a class of phenomena for mea- garding the function and control of the oculomotor surement by the muscle physiologist. However, the system with that for the skeletomotor system, one primary process within the muscle that is modulat- is immediately struck by a disparity. Oculomotor ed by changes in the neurally controlled state of research is characterized by accurate quantitative activation is not length or even tendon strain. Rath- methods, well-defined performance criteria, math- er, it is a complex, statistically distributed set of ematical models of control and feedback regula- forces in the cross-bridges, which are highly depen- tion, and a highly evolved theoretical basis in engi- dent on the direction and magnitude of cross-bridge neering control systems. Skeletomotor research has motion. been and continues to be plagued by phenomenol- It is only in circumstances such as the eyeball and ogical description, intractable, unstable and inap- ear pinna that muscles find themselves controlling propriate models, and an allied field of robotic engi- the unopposed motion of virtually massless, inelas- neering whose greatest contribution continues to be tic objects in a frictionless, low-viscosity medium. proofs of the impossibility of trajectory planning in Under such circumstances, muscles are well de- real time (Loeb, 1983). scribed and easily replaced by simple actuator Of course, it might be argued that the skeleto- mechanisms of the sort commonly employed in motor system is simply a more complicated embod- conventional electromechanical engineering. How- iment of the principles underlying oculomotor con- ever, in more typical skeletomotor configurations, trol, and that the state of knowledge simply lags the addition of large inertial effects, gravity and behind for that reason. However, I shall argue that other external loads, and elastic storage represent the oculomotor system represents an almost sin- not mere complexities, but rather compel the motor gular exception to the more general function and system to adapt entirely different modes of opera- control of striate muscles. Rather than offering a tion. In such systems, one frequently encounters cleaner crucible in which to concoct and test general muscles with highly specialized internal architec- theories of motor control, it has provided a pro- tures to improve performance under such kinemat- tected niche for the preservation of a seductive over- ically diverse conditions as active shortening, active simplification inherited from the study of isolated lengthening, and isometric force generation. The muscles. Muscle physiology research continues to torques and length changes of a given muscle acting be artificially constrained by the undeniable obser- across one joint may depend on the position of the vation that activated muscles contract. If the muscle joint and possibly of other joints spanned by mul- tiarticular muscles. Such actuators are a far cry timized structure for the efficient generation of sus- from the simple torque motors of robotic systems, tained locomotion needed for predatory hunting. so it is not surprising that mechanical engineering This is not to say that such locomotion is the sole has had little to offer in the way of biologically rel- activity of the hindlimb or that it has been entirely evant computational models or control theories. optimized with efficiency as the only goal. Rather In studying the function of any single muscle in this suggests the likelihood that the highly organ- - such a dynamic system, the muscle should not be ized and largely invariant structures to be found in considered directly as a cause of the observed mo- the cat hindlimb derive from real function rather tion; rather, it is better considered as a generator of than evolutionary accident and that this function , an "impulse" added to the system. Impulse is the is likely to be expressed during locomotion. While kinetic contribution to momentum given by the much of the general structure of the cat hindlimb product of force and time. The impulse is the re- is undoubtedly inherited from other, perhaps very sultant of the interaction of the activated cross- different ancestors, the specifics have come to em- bridge sites (bearing calcium and ATP but not body a great wealth of constructive and instructive necessarily attached) with the motion that is largely improvements resulting from the interplay of ran- imparted to the muscle by the inertia of limb seg- dom mutations with their consequences in situa- ments and the action of other forces external to the tions with various probabilities of occurrence (for muscle, including other muscles. As we shall see, discussion, see Partridge, 1982). there is evidence that both the anatomical architec- A fairly complete phenomenology of cat loco- ture and the neurophysiological control circuitry motion has been available in the literature for some for the muscles of locomotion are highly dependent time (see Table I), but it has been only superficially on the particular kinematic conditions under which analyzed biomechanically. Data collection methods each muscle is called upon to provide this impulse. external to the animal (cinephotography and force If there are any single, unifying principles of motor plates) have permitted analysis of the stability of control, they must be expressed in terms that tran- the quadrupedal patterns and extraction of joint scend the concerns local to any such specialized angles and some muscle lengths. The availability of structure as the extraocular muscles, which deal reasonably reliable EMG signals spurred the ob- with only one kinematic condition. servation that at least some muscles tended to be- come active when operating near their optimal The locomotor program in the cat hindlimb lengths, as determined during tetanic electrical stimulation of single muscles in acute preparations The phenomenology of terrestrial locomotion has (Stephens, 1975). However, even more important been thoroughly studied in many species having than the length/tension properties of muscle are the two, four or six legs used in a variety of gait pat- velocity/tension properties (see Joyce et al., 1969). terns. There has been special interest in the cat hind- These have large, nonlinear effects on force output, limb during walking and trotting because of the energetic efficiency, and on the generation of pro- ease with which these gaits are elicited in various prioceptive signals from muscle spindles. Only a p intact and reduced preparations, and the wealth of few muscles having suitable tendon structures have data available on the anatomical and physiological been monitored by surgically implanted force trans- properties of the myoskeletal and neural systems as ducers (Walmsley et al., 1978; O'Donovan et al., they have been studied in this species in isolation 1982; Abraham and Loeb, 1985), and there has over the past century (for reviews, see Grillner, been no quantitative analysis of muscle velocity in 1975; Wetzel and Stuart, 1976). The cat hindlimb any of the kinesiological studies. A further weak- model is a fortuitous choice, because it appears to ness is the absence of a thorough analysis of the represent a highly evolved and thus presumably op- free-body forces operating in this complex open- TABLE I major muscle groups in the cat hindlimb at various points in the walking step cycle (phases from Phil- Phenomenology of cat locomotion lipson, 1905). It is derived from many different ex- perimental sources and from biomechanical model- Quadrupedal locomotion Classical references ing techniques still under development in a colla- studies borative project with Professor William Levine of the Electrical Engineering Department of the Uni- Myoskeletal anatomy Crouch (1969) versity of Maryland (Loeb et al., 1983; Marks et Gait patterns and stride Hildebrand (1966) lengths Arshavsky et al. (1965) al., 1984). The stick figure sequence at the bottom Stuart et al. (1973) represents every second field of a 60 field per second Step cycle phase times Goslow et al. (1973) videotaped walking sequence, with joint positions Miller et al. (1975a,b) determined by a combination of skin markers and Ground forces Manter (1938) trigonometric equations based on post-mortem Joint angles Engberg and Lundberg (1969) Goslow et al. (1973) measurement of limb segments. In each figure at the Muscle lengths Goslow et al. (1973) top, the location of each of eleven major muscle EMG phasing Engberg and Lundberg (1969) groups is shown by a fine line if the muscles are Tokuriki (1973a,b) inactive (or nearly so) and by a spindle-shaped Lengthltension correlate Stephens et al. (1975) thickening if the muscles are generating substantial (incomplete) Muscle forces incomplete - see text amounts of active force (relative to the peak for Muscle velocity not available each muscle in the step cycle as measured by EMG Velocity/tension correlate not available and, in some cases, tendon strain gauges). Each
Details
-
File Typepdf
-
Upload Time-
-
Content LanguagesEnglish
-
Upload UserAnonymous/Not logged-in
-
File Pages8 Page
-
File Size-