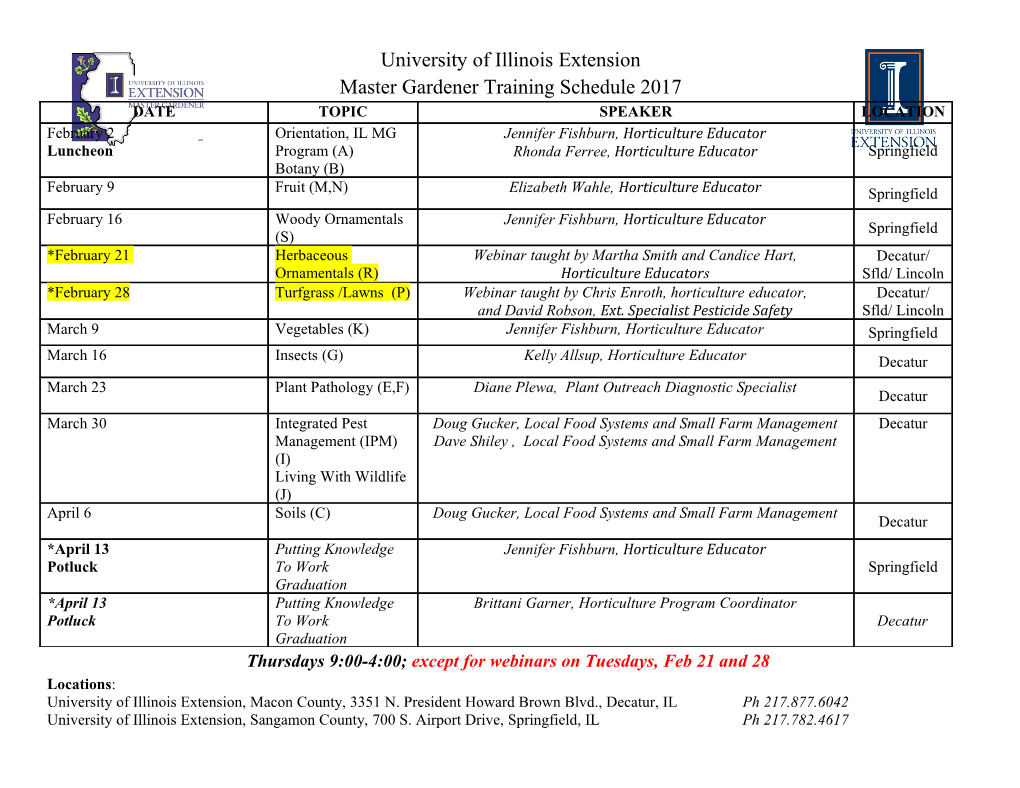
Article Conserved Functions of Ether Lipids and Sphingolipids in the Early Secretory Pathway JIMENEZ ROJO, Noemi, et al. Abstract Sphingolipids play important roles in physiology and cell biology, but a systematic examination of their functions is lacking. We performed a genome-wide CRISPRi screen in sphingolipid-depleted human cells and identified hypersensitive mutants in genes of membrane trafficking and lipid biosynthesis, including ether lipid synthesis. Systematic lipidomic analysis showed a coordinate regulation of ether lipids with sphingolipids, suggesting an adaptation and functional compensation. Biophysical experiments on model membranes show common properties of these structurally diverse lipids that also share a known function as glycosylphosphatidylinositol (GPI) anchors in different kingdoms of life. Molecular dynamics simulations show a selective enrichment of ether phosphatidylcholine around p24 proteins, which are receptors for the export of GPI-anchored proteins and have been shown to bind a specific sphingomyelin species. Our results support a model of convergent evolution of proteins and lipids, based on their physico-chemical properties, to regulate GPI-anchored protein transport and maintain homeostasis in the early secretory [...] Reference JIMENEZ ROJO, Noemi, et al. Conserved Functions of Ether Lipids and Sphingolipids in the Early Secretory Pathway. Current Biology, 2020, vol. 30, p. 3775-3787.e7 DOI : 10.1016/j.cub.2020.07.059 Available at: http://archive-ouverte.unige.ch/unige:141206 Disclaimer: layout of this document may differ from the published version. 1 / 1 1 2 3 4 5 6 7 8 9 10 11 12 13 14 Conserved function of ether lipids and sphingolipids in the early secretory pathway 15 16 Noemi Jiménez-Rojo1,2, Manuel D. Leonetti3, Valeria Zoni4, Adai Colom1, Suihan Feng1, Namrata R. Iyengar5, 17 Stefan Matile6, Aurélien Roux1, Stefano Vanni4, Jonathan S. Weissman3, Howard Riezman1*. 18 19 20 1NCCR Chemical Biology, Department of Biochemistry, University of Geneva, 1211, Geneva, Switzerland. 21 2Biofisika Institute (CSIC, UPV/EHU) and Department of Biochemistry, University of the Basque Country, Leioa, Spain 22 3Department of Cellular and Molecular Pharmacology, University of California, San Francisco, California 94158; Howard Hughes Medical 23 Institute, San Francisco, California 94158. 24 4Department of Biology, University of Fribourg, Fribourg, Switzerland. 25 5Institute of Protein Biochemistry (IBP), Italian National Research Council (CNR), Napoli, Italy. 26 6NCCR Chemical Biology, Department of Organic Chemistry, University of Geneva, 1211, Geneva, Switzerland. 27 28 29 30 31 32 33 34 35 36 37 38 39 40 41 42 43 44 45 1 46 SUMMARY 47 Sphingolipids play important roles in physiology and cell biology, but a systematic examination of 48 their functions is lacking. We performed a genome-wide CRISPRi screen in sphingolipid-depleted 49 cells and identified hypersensitive mutants in genes of membrane trafficking and lipid biosynthesis, 50 including ether lipid synthesis. Systematic lipidomic analysis showed a coordinate regulation of 51 ether lipids with sphingolipids suggesting an adaptation and functional compensation. Biophysical 52 experiments on model membranes show common properties of these structurally diverse lipids that 53 also share a known function as GPI anchors in different kingdoms of life. Molecular dynamics 54 simulations show a selective enrichment of ether phosphatidylcholine around p24 proteins, which 55 are receptors for the export of GPI-anchored proteins and have been shown to bind a specific 56 sphingomyelin species. Our results support a model of convergent evolution of proteins and lipids, 57 based on their physico-chemical properties, to regulate GPI-anchored protein transport and 58 maintain homeostasis in the early secretory pathway. 59 60 61 INTRODUCTION 62 63 The maintenance of membrane lipid homeostasis is an energetically expensive yet 64 necessary process in cells. Lipid diversity has evolved together with cell complexity to give 65 rise to thousands of different lipids species with specific functions, many of which are still 66 unexplored [1, 2]. Moreover, different lipid metabolic pathways are interconnected, and 67 cells show a high phenotypic plasticity when adapting to changes in membrane lipid 68 composition, which makes it difficult to disentangle the function of individual lipid species 69 [3]. A systematic analysis of the cellular responses to perturbation of specific synthetic 70 pathways is thus needed to reveal co-regulated lipid networks and uncover new lipid 71 functions. 72 Sphingolipids (SL) are a class of lipids that contain a sphingoid-base backbone, in contrast 73 to the more commonly found glycerol backbone in glycerophospholipids (GPL). These 74 bioactive lipids have been extensively studied in the last decades, revealing distinctive 75 physico-chemical properties and connections to diseases [4, 5]. SL have been implicated in 76 diabetes [6], cancer [7] and inflammation [8], and mutations in SL synthetic or metabolic 77 enzymes are associated with severe genetic disorders [9-11]. SL species sphingosine (So) 78 and ceramide (Cer) can permeabilize membranes [12, 13]; Cer induces the flip-flop of 79 neighbouring lipids [14] and can phase-separate to form membrane platforms important 80 for signalling [15]. The most abundant SL species, sphingomyelin (SM), has been shown to 81 modulate membrane properties and regulate signalling pathways [16, 17]. Besides direct 82 phosphorylation of ceramide synthases [18-20], the only direct regulators of sphingolipid 83 synthesis identified are Orm proteins (ORMDL in mammalian cells), that associate with 84 serine palmitoyl transferase (SPT), the first enzyme of the sphingolipid de novo synthetic 85 pathway, and mediate the feedback regulation of sphingolipid production in cells [21, 22]. 86 Despite this body of work, many basic aspects of the regulation, molecular interactors and 87 functions of SL still remain poorly understood. 88 2 89 Ether lipids (EL) form a poorly characterized lipid family which represents a subclass of 90 glycerophospholipids that are defined by the presence of an ether bond (or vinyl-ether, for 91 plasmalogens) in lieu of the ester bond found in canonical phospholipids at the sn-1 92 position. This feature, which is intermittently represented across the evolutionary tree, is 93 decisive for the behaviour of these lipids in membranes, and for specific lipid-protein 94 interactions [23]. Interestingly, EL are not only present in membranes as abundant lipid 95 components, where they can range from approximately 5 to 20 mol % of the total GPL 96 content, but are also a major building block of glycosylphosphatidylinositol (GPI) anchors, 97 a specialized protein post-translational modification. Peroxisomal synthesis of EL is 98 necessary for the synthesis of the 1-alkyl, 2-acyl phosphatidylinositol GPI anchor, which is 99 attached to most of the mammalian GPI-anchored proteins (GPI-AP) in the ER [24, 25]. GPI- 100 AP are then exported (through binding to p24 proteins: TMED2 and TMED10 in human) 101 from the ER to the plasma membrane via the Golgi complex, where they undergo different 102 remodelling modifications replacing the unsaturated fatty acid in the sn-2 position with a 103 saturated one. Interestingly, the same p24 proteins that selectively pack GPI-AP into the 104 COPII carriers [26, 27] are also involved in COPI-dependent retrograde transport [28, 29] and 105 p24 proteins bind a particular sphingomyelin species (SM C18 n-acyl) that regulates the 106 initiation of COPI vesicle budding and retrograde transport from the Golgi to the ER [30]. 107 108 Here, we apply high-throughput genetics and lipidomic methods to uncover novel 109 regulatory aspects of sphingolipid metabolism and we find a co-regulation of this lipid class 110 with the structurally unrelated EL. Our multidisciplinary approach combining MD 111 simulations with the study of GPI-AP transport kinetics establishes an active role for 112 (ether)lipids in the transport of GPI-AP from the ER to the Golgi, and emphasizes the 113 physico-chemical properties of EL that enabled them to be selected in mammalian cells to 114 perform this function. 115 116 117 118 RESULTS AND DISCUSSION 119 120 A genetic screen in sphingolipid-depleted cells highlights lipid co-dependencies and 121 uncovers a major role for sphingolipids in intracellular transport 122 To identify pathways regulating cellular responses to sphingolipid depletion, we performed 123 a genome-wide CRISPRi growth screen for genes that modulate sensitivity to myriocin, an 124 inhibitor of de novo sphingolipid synthesis. To ensure dependence on de novo lipid 125 synthesis (as opposed to exogenous lipid intake, which can circumvent the need for cellular 126 synthesis [31]), we cultured human K562 cells in lipid-depleted serum (see Material and 127 Methods). Indeed, we found that myriocin treatment efficiently arrested growth in lipid- 128 depleted media but not in lipid-rich media, while lipid depletion alone did not compromise 129 growth (Fig S1A). Lipidomic analysis verified that myriocin treatment severely decreased 130 cellular sphingolipid levels (Fig 2 A, B, Fig S1 B) under these conditions. 3 131 132 To systematically repress gene expression, we used an ultra-complex genome-wide CRISPRi 133 library targeting 15,977 genes using 10 sgRNAs per gene [32], also including 1,000 non- 134 targeting control sgRNAs to allow precise measurement of experimental noise. For 135 screening,
Details
-
File Typepdf
-
Upload Time-
-
Content LanguagesEnglish
-
Upload UserAnonymous/Not logged-in
-
File Pages41 Page
-
File Size-