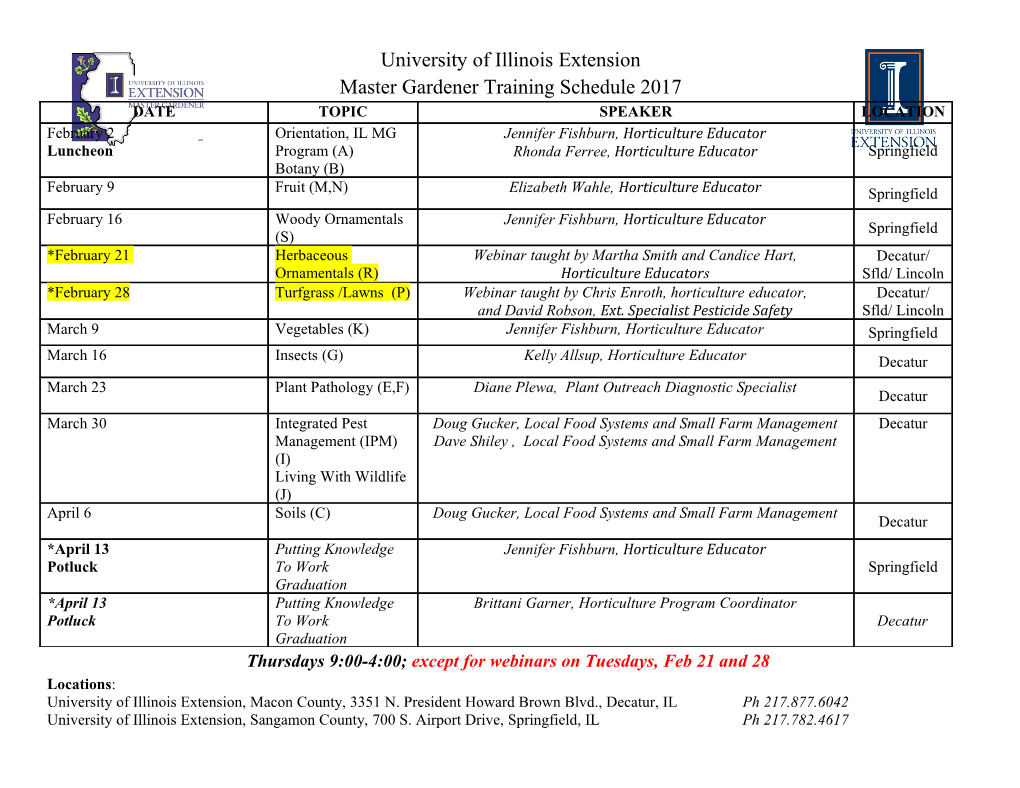
www.nature.com/scientificreports OPEN Performance assessment of total RNA sequencing of human biofuids and extracellular vesicles Celine Everaert1,2,7, Hetty Helsmoortel1,2,7, Anneleen Decock1,2, Eva Hulstaert1,2,3, Ruben Van Paemel 1,2, Kimberly Verniers1,2, Justine Nuytens1,2, Jasper Anckaert1,2, Nele Nijs4, Joeri Tulkens2,5, Bert Dhondt2,5,6, An Hendrix 2,5, Pieter Mestdagh1,2 & Jo Vandesompele 1,2,4* RNA profling has emerged as a powerful tool to investigate the biomarker potential of human biofuids. However, despite enormous interest in extracellular nucleic acids, RNA sequencing methods to quantify the total RNA content outside cells are rare. Here, we evaluate the performance of the SMARTer Stranded Total RNA-Seq method in human platelet-rich plasma, platelet-free plasma, urine, conditioned medium, and extracellular vesicles (EVs) from these biofuids. We found the method to be accurate, precise, compatible with low-input volumes and able to quantify a few thousand genes. We picked up distinct classes of RNA molecules, including mRNA, lncRNA, circRNA, miscRNA and pseudogenes. Notably, the read distribution and gene content drastically difer among biofuids. In conclusion, we are the frst to show that the SMARTer method can be used for unbiased unraveling of the complete transcriptome of a wide range of biofuids and their extracellular vesicles. All human biofuids contain a multitude of extracellular nucleic acids, harboring a wealth of information about health and disease status. In addition to established non-invasive prenatal testing of fetal nucleic acids in mater- nal plasma1, liquid biopsies have emerged as a novel powerful tool in the battle against cancer2. Although in the past most attention was given to circulating DNA, its more dynamic derivate extracellular RNA may provide additional layers of information. However, RNA sequencing in biofuids is technically challenging. Low input amounts, large dynamic range, and (partial) degradation of RNA hamper straightforward quantifcation. While sequencing of small RNAs3 and targeted or capture sequencing of longer RNAs4 proved to be successful, studies using total RNA sequencing on biofuids are rare. To date, only a few whole transcriptome profling attempts were made on urine, plasma or extracellular vesicles5–9, quantifying both polyadenylated and non-polyadenylated RNA transcripts (Table 1). However, all these methods sufer from one or more limitations such as short fragment length, low number of quantifed genes or a high level of ribosomal RNA contamination. Te majority of these methods lack a thorough assessment of data quality under the form of technical repeatability and quantitative accuracy. Te advantages of total RNA sequencing are plentiful. Indeed, detection is not limited to a set of pre-defned targets, nor to (3′ ends of) polyadenylated RNAs. Next to polyadenylated mRNAs, various other RNA biotypes including circular RNAs, histone RNAs, and a sizable fraction of long non-coding RNAs can be distinguished. In addition, the study of posttranscriptional regulation is possible by comparing exonic and intronic reads10. Altogether, this generates a much more comprehensive view of the transcriptome. Here we aimed to assess the performance of a strand-specifc total RNA library preparation method for dif- ferent types of biofuids and derived extracellular vesicles (EVs). We applied the method on platelet-rich plasma, platelet-free plasma, urine and conditioned medium from human healthy donors, cancer patients or cancer cells grown in vitro. More specifcally, the SMARTer Stranded Total RNA-Seq Kit – Pico Input Mammalian, including a ribosomal RNA depletion step at the cDNA level, was extensively evaluated. We found the method to be accurate 1Center for Medical Genetics, Department of Biomolecular Medicine, Ghent University, Ghent, Belgium. 2Cancer Research Institute Ghent, Ghent, Belgium. 3Department of Dermatology, Ghent University Hospital, Ghent, Belgium. 4Biogazelle, Zwijnaarde, Belgium. 5Laboratory of Experimental Cancer Research, Department of Human Structure and Repair, Ghent University, Ghent, Belgium. 6Department of Urology, Ghent University Hospital, Ghent, Belgium. 7These authors contributed equally: Celine Everaert and Hetty Helsmoortel. *email: [email protected] SCIENTIFIC REPORTS | (2019) 9:17574 | https://doi.org/10.1038/s41598-019-53892-x 1 www.nature.com/scientificreports/ www.nature.com/scientificreports library prep biotypes small total reference method RNA RNA sample types strandedness contaminants mRNA lncRNA microRNA circRNA Ion Total RNA-seq v2 EVs from serum and Li et al., 2014 + − stranded 2–50% rRNA + − + − (Termofsher) urine Qin et al., 2015 TGIRT-seq − + plasma (1 mL) stranded 0.9–6.3% rRNA + + + − Savelyeva et al., SOLiD sequencing blood, plasma, EVs − + NA 4–33% rRNA + + + − 2017 technology from plasma Amorim et al., Ion Total RNA-seq v2 + − EVs from plasma stranded NA + + + − 2017 (Termofsher) high YRNA and Giraldez et al., rRNA content 2019 Akat et phospho-RNA-seq + − plasma, bone marrow NA + + + − (percentage not al., 2019 reported) Zhou et al., SILVER-seq − + serum (droplet) NA + + + − 2019 plasma, urine, mitochondrial rRNA this study SMARTer-seq − + conditioned medium stranded depending on sample + + − + (200 µl) and EVs type Table 1. Comparison of RNA-seq methods to study biofuids and extracellular vesicles. and precise. Low-input volumes are technically feasible and the method allows the detection of several thousand genes of diferent classes. Results Read distribution drastically difers among biofuids. In a frst experiment (Fig. 1A), we sequenced platelet-rich plasma (PRP) and platelet-free plasma (PFP) from two diferent healthy donors. We collected blood in EDTA tubes, hence the ‘e’ in front of ePRP and ePFP throughout the manuscript. From each plasma fraction, two technical RNA extraction replicates were performed, resulting in four sequenced samples per donor. Because of the low input, between 53.0% and 88.2% of the reads were PCR duplicates (Sup Fig. 1). PCR duplicates arise when multiple PCR products from the same original template molecule bind to the sequencing fow cell. For better quantitative accuracy, we removed the duplicates for further analysis. Te variation in PCR duplicate levels between plasma fractions is related to the amount and quality of input RNA. As we will illustrate below, ePRP has a higher RNA input concentration, which explains the lower number of duplicate reads compared to ePFP. Afer duplicate removal we mapped the remaining (deduplicated) reads to the reference genome (Fig. 2A). Four catego- ries of reads can be distinguished here: uniquely mapping reads, multi-mapped reads aligning to several genomic positions, reads that are too short to map, and unmapped reads. Te number of unmapped and multi-mapped reads was similar between plasma with and without platelets. However, ePFP samples contain much more reads that are too short to map. As a consequence, ePRP contains approximately twice as many uniquely mapped reads, possibly the result of more intact RNA in platelets. However, when only considering these unique reads, more than 75% of them derived from mitochondrial RNA (mtRNA) in ePRP (Fig. 2B). In contrast, ePFP contains at least three times less mtRNA and considerably more reads mapping to nuclear DNA. Finally, also the distribution between exonic, intronic and intergenic reads difers between platelet-rich and platelet-free plasma (Fig. 2C). In the second experiment (Fig. 1B), we sequenced conditioned medium from breast cancer cells (CM), platelet-free plasma from a third healthy donor collected in a citrate blood collection tube (cPFP) and urine from a prostate cancer patient. In addition, we purifed EVs from these three fuids and performed extensive quality control using western blot, electron microscopy and nanoparticle tracking analysis (Sup Fig. 2). We sequenced the EV samples together with their fuids of origin. For this experiment, two technical replicates were introduced at the level of library preparation for each condition, resulting in 12 libraries. Because only one biological sample of each biofuid was included in this experiment, we should be cautious when generalizing diferences among biofuids. With the exception of plasma, the number of PCR duplicates is lower in EVs compared to their paren- tal biofuid (Sup Fig. 1). As mentioned earlier, the levels of PCR duplicates are typically lower in samples with higher input quality and concentration. But, as we will see in the next paragraph, RNA input amounts in EVs are not higher compared to their fuid of origin. Other explanations, at least in part, could be the protective efect lipid bilayers have on the quality of their RNA cargo and the wide diferences in RNA content. Interestingly, also mapping rates can difer substantially among biofuids and/or their EVs (Fig. 2A). In our setup for instance, the fraction of unique reads ranges from 7.69% in cPFP EVs to 90.2% in EVs isolated from conditioned medium. When looking at the mapping properties of the unique reads, almost all samples mainly contain reads that map to nuclear DNA (Fig. 2B). Only platelet-free plasma contains 25.8% mitochondrial RNA, comparable to the per- centages that were generated in the healthy donors of the frst experiment. Lastly, most reads mapping to nuclear DNA are exonic. Te only exception here are cPFP EVs that contain a larger fraction of intronic and intergenic reads (Fig. 2C). While the platelet-free plasma samples in the frst and second experiment seem
Details
-
File Typepdf
-
Upload Time-
-
Content LanguagesEnglish
-
Upload UserAnonymous/Not logged-in
-
File Pages16 Page
-
File Size-