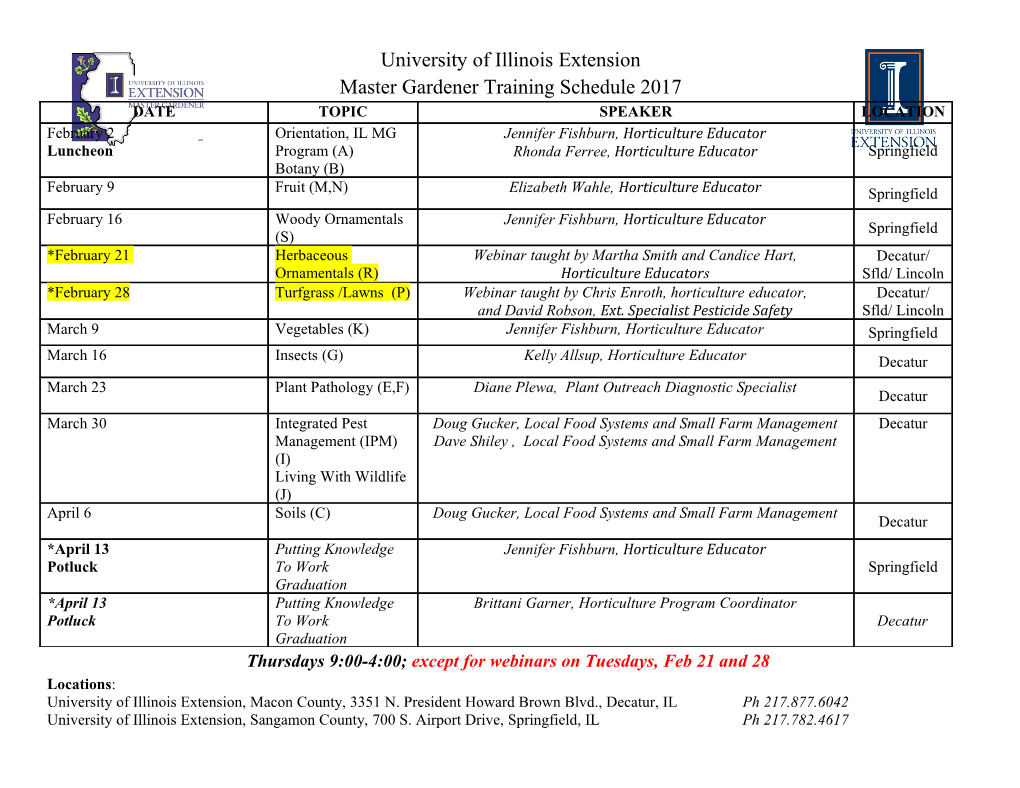
Appendix 9: National-scale responses of river macroinvertebrates species to changes in temperature and precipitation Abstract Global climate change is expected to have a large impact on the biodiversity and functioning of freshwater ecosystems because of shifts in temperature, seasonality and weather. The response of freshwater organisms to climate change is likely to vary according to their environmental optima, with some species thriving under new conditions, while some at risk species may decline in abundance. These changes could significantly alter biodiversity, trophic interactions and key ecological processes, affecting current and future management and conservation regimes, as well as compliance with current environmental legislation such as the Water Framework Directive. This study examines the response of 137 river macroinvertebrate species to two climatic variables (temperature and precipitation) from 1,588 sampling sites across the United Kingdom over 15 to 25 years (1983-2007). Using a bespoke modelling method, the sensitivity of each species to changes in temperature and precipitation was identified, with the aim of inferring likely changes in the abundance of particular species in response to climate change. The characterisations of species responses are also used to demonstrate that a combination of species-specific traits and environmental preferences may be a systematic way to predict impacts. Introduction Freshwater ecosystems are considered one of the richest ecosystems globally in terms of biodiversity, sustaining a disproportionate high fraction of species per surface area relative to other ecosystems (Dudgeon et al., 2006, Balian et al., 2008). This biodiversity supports a range of important ecosystem processes (Woodward, 2009) , many of which provide key goods and services, such as the supply of clean drinking water, the dilution of pollution and the harvest of fish and other produce, to name but a few (Millennium Ecosystem Assessment, 2005). Despite their inherent value and importance, freshwater ecosystems are especially susceptible to degradation and climate change (Hart & Calhoun, 2010, Ormerod et al., 2010), manifesting in freshwater biodiversity declining at a much faster rate than either terrestrial or marine ecosystems (Ricciardi & Rasmussen, 1999, Sala et al., 2000, Jenkins, 2003, Heino et al., 2009). Stream and rivers, particularly, rank among the most threatened freshwater networks owing to the combined effects of multiple pressures. These include warming temperatures, increased frequency of extreme hydrological fluctuations, habitat destruction and fragmentation, alien species invasion and point and diffuse pollution (Malmqvist & Rundle, 2002, Vorosmarty et al., 2010). Reduced biodiversity may disrupt the functioning of ecosystems, threatening their intrinsic resilience to change (Loreau et al., 2001, Hooper et al., 2005), which may directly impact the ecosystem services on which human communities rely (Strayer & Dudgeon, 2010). Evidence that climate change is occurring and impacting freshwater biodiversity is now unequivocal (IPCC, 2013), with increasing vulnerability projected for the future due to the interaction of climatic stressors (temperature, precipitation) with other stressors such as pollution and habitat loss (Domisch et al., 2013, Floury et al., 2013, Khamis et al., 2014). Any increase in air temperature is likely to translate directly into warmer water temperatures (Mohseni & Stefan, 1999, Morrill et al., 2005). In line with this, the temperatures of flowing waters have risen in Europe. For example water temperature in the Danube has increased by up to 1.7 oC since 1901 (Webb & Nobilis, 2007), and 1 temperature has increased by 2.6 oC in French rivers between 1979 and 2003 (Daufresne & Boet, 2007), and by 1.4 oC in Welsh streams between 1981 and 2005 (Durance & Ormerod, 2007). Warmer temperatures are likely to change species distributions, growth rates and phenology (Root et al., 2005, Friberg et al., 2009), in turn affecting food web dynamics and ecosystem processes (Kishi et al., 2005). Water quality may decrease as microbial activity and decomposition of organic matter increase, aggravating the reduced dissolved oxygen levels associated with higher temperatures. Aquatic species unable to migrate (regionally to cooler climes or within a river to the cooler headwaters) may face local extinctions. Conversely, there is a strong risk that non native invasive species, with broader temperature tolerances, may spread to new territories and establish themselves rapidly, applying further stress to native species. (Poff et al., 2002, Rahel & Olden, 2008). Climatic changes to air and water temperature will cause shifts in the timing and intensity of precipitation and changes in the rates of evapotranspiration. Because these affect the volume and timing of runoff, and modify groundwater recharge, changes to the hydrology of freshwater systems are expected. These include a greater frequency, intensity and duration of extreme events such as storms/floods and droughts, increased peak flows and reduced base flows (IPCC, 2007) . These changes mediated by the supply and the quality of water, when combined with higher water temperature and further anthropogenic stressors, make freshwater ecosystems amongst the most vulnerable to climatic change (Allen & Ingram, 2002). Benthic macroinvertebrates are one of most common indicators for biomonitoring the health of lotic ecosystems (Wright et al., 1993, Friberg et al., 2011) and are used in the United Kingdom (UK) and elsewhere to assess compliance with environmental regulations such as the Water Framework Directive (WFD) (European Commission, 2000). Macroinvertebrate communities are known to respond strongly to water temperature (Hawkins et al., 1997, Caissie, 2006), flow alterations (Poff & Zimmerman, 2010) and extreme drought/flood events (Ledger et al., 2013b), therefore provide an ideal system for the study of climate change impacts (Wilby et al., 2010). Three relatively consistent results from studies on macroinvertebrate responses to metrics of a changing climate are (i) alterations in the timing and duration of life cycle phases, such as pupation and emergence periods(Kotiaho et al., 2005, Leberfinger et al., 2010), (ii) the losses of species and trophic interactions, especially predators (Ledger et al., 2013a), and (ii) the geographical distribution of biota, such as shifts in altitudes according to thermal tolerances (Daufresne et al., 2003, Hering et al., 2009) However, the results of most studies are difficult to extrapolate at regional and national scales because they are often constrained to the analysis of macroinvertebrate data in specific habitat types (Zivic et al., 2014) or specific catchment (Daufresne et al., 2003, Durance & Ormerod, 2007) that usually have unique local stressors other than climate. These (e.g. nutrient pollution, oxygen concentrations) may exacerbate, reduce or offset the direct influence of climate change, making it harder to detect (Floury et al., 2013, Vaughan & Ormerod, 2014). For the purpose of improving conservation and management plans, and the prioritisation of interventions and mitigation measures, a better understanding of the sensitivity of macroinvertebrate communities to climate change is necessary at regional or national scales. Despite their advantages to national management programmes, large-scale or regional studies are often limited to the analysis of macroinvertebrate data at a higher level of biological organisation than species, e.g. family level (Floury et al., 2013, Vaughan & Ormerod, 2014). As a result, few studies have examined differences in the responses of individual species within the same taxonomic 2 groups, across a wide range of taxa. Intra-group heterogeneity in species traits (e.g. ecological preferences and life cycle events), and interactions between these traits, may mask contrasting or stronger species responses to climate that are not observed at the higher group level (Hering et al., 2009, Tierno de Figueroa et al., 2010, Conti et al., 2014). This study presents the first comparative assessment of climatic sensitivity using the most comprehensive dataset of lotic macroinvertebrate species abundances, comprising 23 orders, across the UK. A bespoke modelling approach developed in Appendix 2 was used, where the annual spring population abundance of 137 species were modelled as a function of metrics describing local monthly mean air temperature and precipitation. A broad-scale approach was adopted, focusing on evidence for systematic trends across multiple sites over 15-25 years, while excluding any linear trends that may be explained by alternative stressors. The modelling approach proposed in this study assumes that (i) the response of species population abundances to local climate varies throughout the 12 months prior to spring sampling, and is captured by a single oscillating pattern, and (ii) species abundance is likely to be influenced by the local climate in the preceding three years, necessitating the inclusion of a decaying lagged effect. Once models were calibrated, statistically significant relationships were examined and species responses were used to classify any observable trends according to each species’ traits. Model outputs yield a measure of directional change that incorporates month on month local climatic effects on species population abundance, providing a tool to assess the future impact of climate change (e.g. increases in temperature or precipitation)
Details
-
File Typepdf
-
Upload Time-
-
Content LanguagesEnglish
-
Upload UserAnonymous/Not logged-in
-
File Pages29 Page
-
File Size-