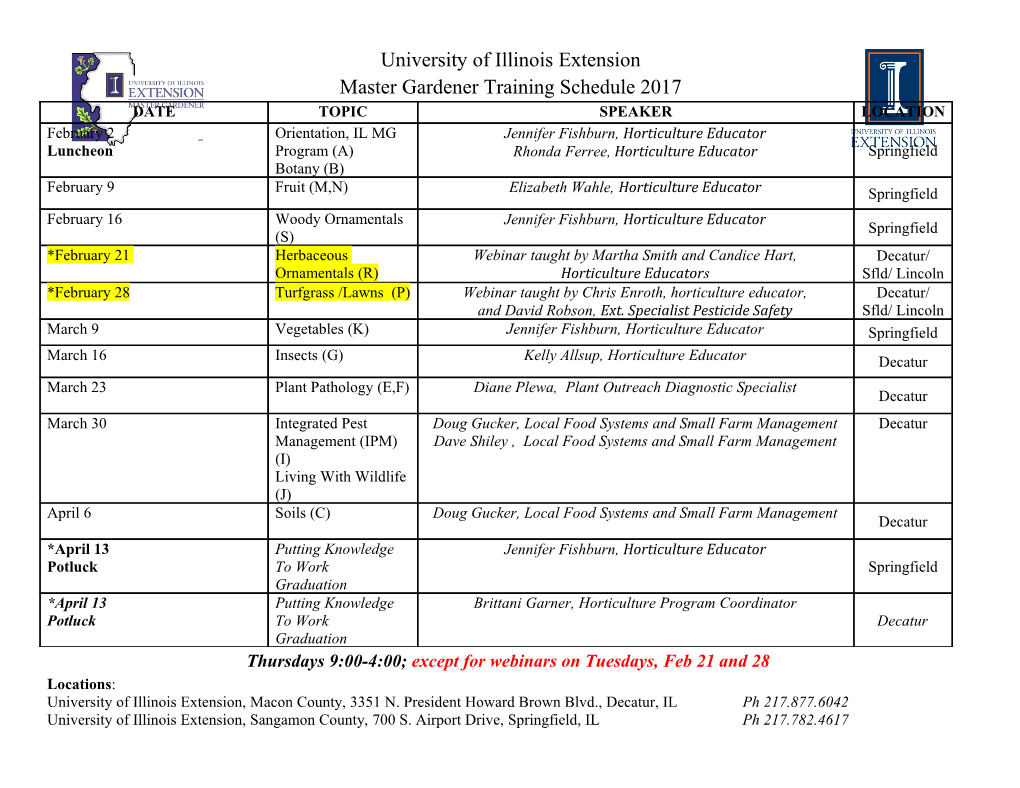
Pro gradu -tutkielma Teoreettinen fysiikka Particle physics models with four generations Hanna Grönqvist 2012 Ohjaaja: Prof. Katri Huitu Tarkastajat: Prof. Katri Huitu Prof. Paul Hoyer HELSINGIN YLIOPISTO FYSIIKAN LAITOS PL 64 (Gustaf Hällströmin katu 2) 00014 Helsingin yliopisto 2 Contents I Perliminaries 5 1 Motivation 7 1.1 Historical overview – the ‘discovery’ of the standard model............... 7 1.2 Problems of the standard model solved by a fourth generation............. 10 1.2.1 Naturalness ................................... 12 1.2.2 Flavor democracy – a solution to the naturalness problem ........... 14 1.2.3 Electroweak Precision Data . ...... 15 1.3 Unitarity constraints on the fourth generation . ............... 17 2 The Standard Model 21 2.1 Quantum chromodynamics . ...... 21 2.1.1 Gauge fixing and Faddeev–Popov ghosts . ....... 22 2.1.2 Renormalization and asymptotic freedom . ......... 24 2.2 Local SU(2) and U(1) symmetries ............................ 25 2.2.1 Isospinsymmetry............................... 25 2.2.2 Quantum electrodynamics . ..... 26 2.3 Spontaneous symmetry breaking . ........ 26 2.3.1 TheHiggsmechanism ............................. 27 2.4 The Glashow–Weinberg–Salam model . ........ 29 2.5 Quark and lepton mixing . ..... 31 2.5.1 Thequarksector ................................ 31 2.5.2 Beyond the SM: mixing in the lepton sector . ....... 33 II The minimal four–generation model 39 3 Phenomenology of the fourth family 41 3.1 Mixing of the fourth family with the first three ones . ............. 41 3.1.1 Sources for constraints . ...... 41 3.1.2 Possible parameter space . ...... 43 3.2 Higgs production and partial decay widths . ............ 47 3.2.1 Higgs production at hadron colliders . ......... 47 3.2.2 Branching fractions . 51 3.2.3 Searches for the Higgs and the fourth family . 53 3 4 CONTENTS 4 Experimental searches 57 4.1 Search strategies – SM4 quarks . ........ 57 4.1.1 General event topologies . ...... 57 4.1.2 Pair production of fourth family quarks . ......... 58 4.1.3 Single production of fourth family quarks . .......... 59 4.1.4 Current experimental bounds . ...... 64 4.2 Searches for fourth generation leptons . ............ 66 4.2.1 Neutrinos and Higgs at the LHC . 67 4.2.2 Searches at future lepton colliders . .......... 68 4.2.3 Existing experimental constraints . .......... 70 4.3 Higgssearches................................... ..... 71 4.3.1 Higgs searches at Tevatron . ..... 71 4.3.2 Higgs searches at the LHC . 72 5 Experimental exclusion of the minimal SM4 75 III The two Higgs doublet model 79 6 Theory of the 2HDM 81 6.1 Why add another doublet? . ...... 81 6.2 The scalar potential and the field content . ........... 82 6.3 Flavorconservation.. .. .. .. .. .. .. .. .. .. .. .. .. ....... 83 6.4 Yukawa couplings of the type II 2HDM . ........ 84 7 4F2HDM phenomenology 85 7.1 Higgs production and decay in the 2HDM–II . ......... 85 7.1.1 Production and decay of the neutral pseudoscalar A0 .............. 86 7.1.2 Production and decay of the light neutral Higgs h0 ............... 88 7.1.3 ThechargedHiggs ............................... 89 7.2 Searches for the 4F2HDM at the LHC . ...... 91 7.2.1 Fourth generation quarks masses . ....... 91 8 Conclusions 95 Appendices 97 A Definitions of electroweak observables 99 B The Goldstone model 101 C Mathematicaldescriptionofneutrinooscillations 105 D Oblique electroweak parameters 107 E Feynman diagrams for production of fourth family particles 113 F Form factors for A0, h0 branching ratios 117 Bibliography 119 Part I Perliminaries 5 Chapter 1 Motivation In this chapter we will shortly review the historical development of the Standard Model of elementary particle physics. The aim of the historical overview is to give an idea as to how the current theory was born, from theoretical predictions and experimental verification with many unexpected discoveries leading to reformulations and expansions of the theory. With this section we wish to show the reader that there have been unexpected events along the way: that there might still be many surprises in store for us and that it might be premature to think that our current view of particle physics is complete. In the second part of this chapter we list some shortcomings of the current model, called the ‘standard model’, and give some arguments in favor of adding a fourth family of particles. Ideas presented in this section will be considered in some detail later on in the text. 1.1 Historical overview – the ‘discovery’ of the standard model The observable matter of the universe consists of atoms that are made up of protons, neutrons and electrons. All of these particles were discovered in the 1930’s, and at the time they were deemed to be elementary constituents, just as had been thought of atoms before that. Before this the photon had also been found, the photon being the quantum of the electromagnetic field that ‘binds’ the electron to the atomic nucleus. The force binding the protons and neutrons of the nucleus was thought to be mediated by a particle called the ‘pi-meson’, as suggested by Yukawa [1]. When Pauli predicted the existence of one more particle, the electron neutrino, as a solution to a problem (missing energy in beta decays) [2], it appeared that a complete picture of particle physics had been established. The situation changed when the muon was discovered some years later, in 1937 [3, 4]. The muon seemed identical to the electron, but with 200 times its mass. It was also very puzzling that this new particle seemed to be produced by strong interactions but its interactions with matter were electromagnetic. A famous physicist, Rabi, then made a comment conveying the amazement at this surprise, cited many times since: ‘Who ordered that?’ [5]. Ten years later the charged pion, predicted by Yukawa, was found [6, 7, 8]. At the same time another meson was discovered, too [9]. Later it became clear that this was similar to Yukawa’s particle, but neutral, and so it became known as the ‘neutral pion’ [10]. These new pieces fit into a beautifully simple theory where electromagnetic interactions are mediated by photons, strong interactions by π-mesons and weak interactions are described by the Fermi four-fermion interaction; all nuclei are bound states of protons and neutrons, atoms bound states of nuclei and electrons and 7 8 CHAPTER 1. MOTIVATION the matter fields classified as leptons (electron and its neutrino), mesons (the pions) and baryons (consisting of protons and neutrons). The beginning of a new decade, the 50’s, opened a new ‘era’ of elementary particle physics [5, 11]: In 1951, cloud chamber observations showed a new neutral particle (Λ), which decayed into a proton and a negative pion; in 1952-1953 the negatively charged Ξ and the charged Σ-particles were detected in cosmic rays at high altitudes. All of these (later called hyperons) were heavier than the known nucleons and exhibited an unusual feature: they were produced relatively often in nuclear collisions, but then they decayed with long life-times of (10 10) (10 8) s, suggesting O − − O − that they were produced in strong interactions but decayed through weak ones. These particles, called ‘strange’ and always produced in pairs led to the discovery of the strange quark [12, 13, 9]. A theoretical explanation was given both by Nakano and Nishijima [14] in Japan, and independently Gell-Mann [15] in the USA. As a part of this explanation they introduced a new quantum number called ‘strangeness’, related to other, already established, quantum numbers. In the following years there was a great accumulation of data on baryon and meson resonances as a consequence of the completion and running of several particle accelerators. By the mid- 1960’s it was realized that the contemporary theory did not suffice as an explanation for the many new particles that were being discovered. The idea of quarks as the constituents of mesons and baryons was put forward by Gell-Mann [16] and Zweig [17] in 1964. Further the notion of ‘color charge’ was introduced in 1964 by Greenberg [18] and in 1965 by Nambu and Han [19]. Since leptons were observed to have a specific pattern, emanating from the discovery of the muon and muon neutrino [20], a fourth quark was predicted in 1964 by Bjorken and Glashow [21], an idea that was further worked on by Glashow, Iliopoulos and Maiani [22] in 1970. It was recognized that the fourth quark allowed for a theory with flavor conserving neutral currents, but not flavor changing ones (called the ‘GIM’ mechanism), as explained by two generations related through Cabibbo mixing [23]. This model was consistent with observations and the fourth quark, called ‘charm’, was found in 1974 [24, 25, 26, 27]. By this time a renormalizable gauge theory had been developed [28, 29, 30, 31], based on the two ‘generations’ of particles observed thus far, the first generation being the u and d quarks, the electron and its neutrino and the second generation consisting of the c and s quarks, the muon and its neutrino. A quantum field theory of strong interactions was formulated in 1973, first suggested by Fritzsch, Gell-Man and Leutwyler [32]. Quarks were deemed to be real particles (as opposed to mere mathe- matical tool) carrying a charge of their own – the ‘color’ charge. In this theory of color interactions, called quantum chromodynamics (QCD), the massless quanta of the color field were named gluons. A very peculiar feature of the theory was discovered not long afterwards – a property later called ‘asymptotic freedom’, needed to describe observed properties of the proton [33, 34, 35, 36, 37]. As had been the case for the theory based on just one family of particles, the theory based on two families suddenly experienced severe problems with the experimental discovery of the tau lepton in 1975 [38]. Two years later, in 1977, a fifth quark, named ‘bottom’, was discovered [39, 40].
Details
-
File Typepdf
-
Upload Time-
-
Content LanguagesEnglish
-
Upload UserAnonymous/Not logged-in
-
File Pages127 Page
-
File Size-