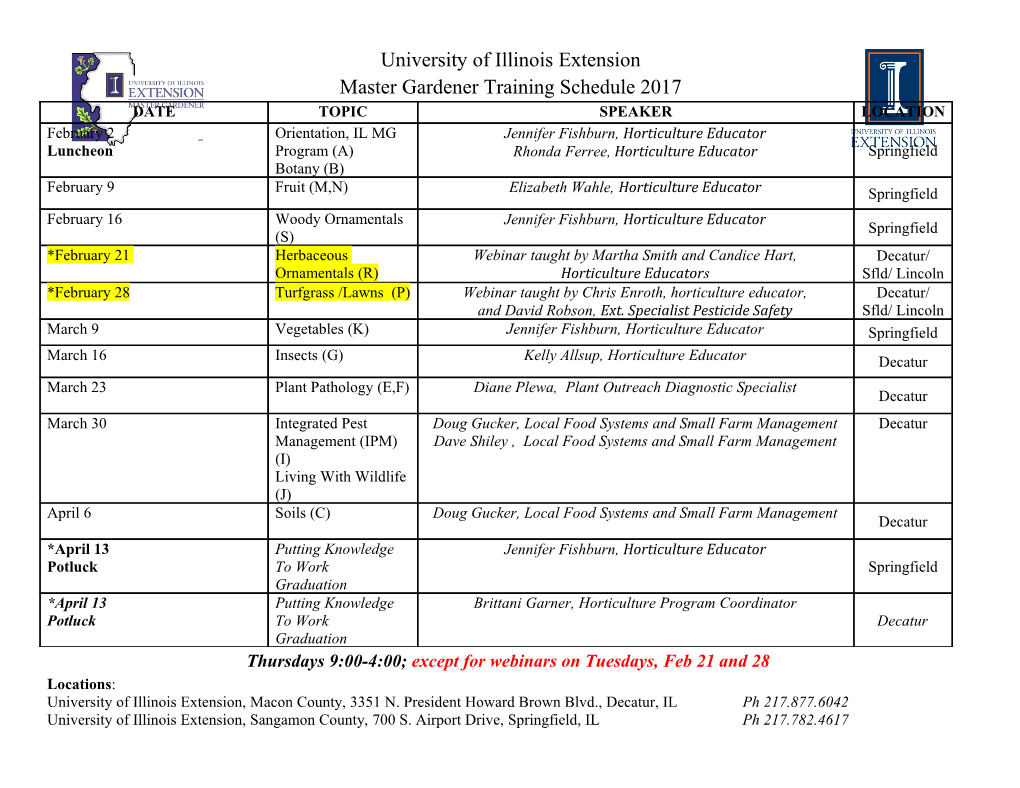
A Review on Transient Thermal Management of Electronic Devices John Mathew Department of Mechanical Engineering, Much effort in the area of electronics thermal management has focused on developing Indian Institute of Technology Bombay, cooling solutions that cater to steady-state operation. However, electronic devices are Mumbai 400076, India increasingly being used in applications involving time-varying workloads. These include e-mail: [email protected] microprocessors (particularly those used in portable devices), power electronic devices such as insulated gate bipolar transistors (IGBTs), and high-power semiconductor laser Downloaded from http://asmedigitalcollection.asme.org/electronicpackaging/article-pdf/144/1/010801/6737531/ep_144_01_010801.pdf by guest on 27 September 2021 Shankar Krishnan1 diode arrays. Transient thermal management solutions become essential to ensure the performance and reliability of such devices. In this review, emerging transient thermal Department of Mechanical Engineering, management requirements are identified, and cooling solutions reported in the literature Indian Institute of Technology Bombay, for such applications are presented with a focus on time scales of thermal response. Mumbai 400076, India Transient cooling techniques employing actively controlled two-phase microchannel heat e-mail: [email protected] sinks, phase change materials (PCM), heat pipes/vapor chambers, combined PCM-heat pipes/vapor chambers, and flash boiling systems are examined in detail. They are com- pared in terms of their thermal response times to ascertain their suitability for the ther- mal management of pulsed workloads associated with microprocessor chips, IGBTs, and high-power laser diode arrays. Thermal design guidelines for the selection of appropriate package level thermal resistance and capacitance combinations are also recommended. [DOI: 10.1115/1.4050002] 1 Introduction microelectronic devices seldom operate under steady-state condi- tions. Figures 1(a) and 1(b) demonstrates the temporal variation in As electronic devices continue to undergo intensifying minia- CPU workloads of a D2461 Siemens—Fujitsu server. The power turization [1,2], power densities persist in being on a rising trend. consumption of the 4 GHz AMD Athlon 64 dual-core processor Heat fluxes over 102 W/cm2 are currently generated by microproc- can be seen to vary continuously with time as it performs an essors [3]. Moreover, hot spots in chips involve localized heat online music search and download operation [40]. fluxes of 1 kW/cm2 or more, leading to excessive local tempera- Another development in the microelectronic industry is the tures [4,5]. Rising user demand for augmented computational per- growing shift toward portable handheld devices such as smart- formance and functionalities has been fueling the development of phones and tablets [41,42]. These devices exhibit long durations high-power microprocessors. Apart from IC devices, power semi- of low-power consumption for regular applications and short conductor devices such as insulated gate bipolar transistors durations of high-power consumption during process-intensive (IGBTs) and laser diode arrays also generate heat fluxes above applications such as video calling/recording [43–45]. 1 kW/cm2 [6–9]. Operation at excessive temperatures impairs Compared to microprocessor chips, a more pronounced tran- device performance and reliability and ultimately causes their fail- sient thermal behavior is exhibited by power semiconductor devi- ure. The different failure modes of microelectronic devices, ces. Rapid fluctuations in junction temperatures are experienced namely mechanical, electrical, and corrosion, are linked to high during high-frequency switching operations. Highly transient ther- operating temperatures [10]. Pecht and Gu discussed the various mal characteristics are also witnessed in high-power semiconduc- failure mechanisms identified in electronic products. Fatigue due tor laser diode arrays [46]. to temporal temperature oscillations leads to failure at locations Cooling solutions that work well under steady-state conditions such as the die-attach, wire bonds, solder leads, bond pads, and may not offer desired thermal characteristics when devices are vias [11]. Similarly, in the case of power electronic devices, high- operated under transient workloads. The primary focus of steady- frequency switching operation results in periodic temperature state cooling systems is to reduce the overall thermal resistance swings. The resulting thermomechanical stresses lead to the fail- (R) of the package so that maximum power can be dissipated ure of the constituent semiconductor components and thereby without exceeding the maximum temperature limit (T ) of the undermine device reliability. Transient thermal management tech- j,max device. The thermal capacitance of the package (C), though, is not niques that reduce temperature swings, for such applications considered. Such a policy of minimizing R without optimizing C involving time-varying power loads, therefore become inevitable. results in the following drawbacks: There has been considerable progress in implementing various cooling techniques to address the thermal management challenges (1) Highly efficient compact cooling devices with low R usu- associated with high heat flux devices. A summary of these tech- ally have low C [47,48]. Packages with low C exhibit sud- niques is listed in Table 1. As can be inferred, developments in den changes in temperature in response to pulsed single-phase, two-phase cooling, jet impingement, and spray cool- workloads. The consequent thermal fatigue effects can be ing have enabled the dissipation of ultrahigh heat fluxes detrimental to device reliability. [9,23,34,35,38,39]. (2) Steady-state cooling systems developed based on Tj,max is While much research work in conventional thermal manage- often over-designed as the average heat dissipation rates of ment deals with cooling under steady-state device operation, most electronic devices are usually lower during operation. (3) Any attempt to augment device performance, for a package 1Corresponding author. designed with a specific R, is constrained by power limita- Contributed by the Electronic and Photonic Packaging Division of ASME for tions governed by Tj,max. publication in the JOURNAL OF ELECTRONIC PACKAGING. Manuscript received September 22, 2020; final manuscript received January 22, 2021; published online The limitations of steady-state-based cooling systems under August 6, 2021. Assoc. Editor: Ercan Dede. pulsed workloads were demonstrated by Jankowski and Journal of Electronic Packaging Copyright VC 2022 by ASME MARCH 2022, Vol. 144 / 010801-1 Table 1 List of conventionally employed thermal management techniques and their performance capabilities as reported in literature Maximum h Cooling Maximum q00 (W/m2K)/Minimum R technique Configuration Authors Coolant (W/cm2) (K/W) Single-phase Remote Tuckerman and Pease Water 790 __/0.09 microchannel [12] Remote Prasher et al. [13] Water 58.33 __/0.41 Remote Lee et al. [14] Water – 22,000/__ Remote Kosar and Peles [15] Water 167 55,000/__ Remote Colgan et al. [16] Water 500 200,000/__ Remote Wang et al. [6] Water 13.64 __/0.006 Ethylene glycol/water 13.64 __/0.003 Embedded Zhou et al. [9] Water 127.5 64,000/__ Downloaded from http://asmedigitalcollection.asme.org/electronicpackaging/article-pdf/144/1/010801/6737531/ep_144_01_010801.pdf by guest on 27 September 2021 Two-phase cooling Remote Kosar et al. [17] R-123 312 68,000/__ (forced convection in Remote Zhu et al. [18] Water 969 210,000/__ microchannels) Remote David et al. [19] Water 82 300,000/__ Remote Fazeli et al. [20] Water 380 260,000/__ Remote Kandlikar et al. [21] Water 506 193,000/__ Embedded Wang et al. [6] R134a 13.64 29,000/0.003 Embedded Green et al. [22] Air/FC-72, water 100, 1000 (hot spot) __/__ Embedded Drummond et al. [23] HFE-7100 910 28,200/ Two-phase cooling Pool boiling Li and Peterson [24] Water 350 __/__ (natural convection) Pool boiling Rahman et al. [25] Water 230 210,000/__ Pool boiling Jaikumar and Kandli- Water 394 713,000/__ kar [26] Thermosyphon Raju and Krishnan Water, water-sodium 31.3 52,000/__ [27] lauryl sulfate surfactant Jet impingement Ndao et al. [28] R134a 275 150,000/__ cooling Rau et al. [29] HFE-7100 205.8 19,000/__ Joshi and Dede [30] R-245fa 218 97,800/__ Wu et al. [31] Water 160 41,377/__ Capillary-fed boiling Palko et al. [32] Water 1280 630,000/__ Spray cooling Pautsch and Shedd FC-72 77.8 13,000/__ [33] Fabbri et al. [34] Water 93 25,000/__ Mudawar et al. [35] HFE-7100 263 __/__ Tan et al. [36] R134a 165 39,000/__ Zhou et al. [37] R410a 330 300,000/__ McCluskey [48], who examined the transient thermal response of 2 Emerging Transient Thermal Management a semiconductor power electronics package when cooled using Requirements five different configurations (cases 1–5 as illustrated in Fig. 2(a)). The overall thermal resistances (R) and thermal capacities (C)of The transient thermal issues associated with different micro- the configurations were in decreasing orders of their extents of electronic devices vary in terms of their operating conditions and cooling integration. As seen from Fig. 2(b), the maximum temper- the time scales involved. In this section, the transient thermal atures of cases 3 and 5 were, respectively, 30% and 207% higher management challenges associated with microprocessor chips, than that of case 1, while their temperature swings were higher by IGBTs, and high-power laser diode devices are examined in 106% and 401% as the period of the pulses approached their ther- detail. mal time constants (s ¼ RC). From this study, it is evident that highly efficient steady-state cooling solutions with low thermal 2.1 Microprocessors. While the ongoing trend toward dimin- resistances (R) and thermal capacities (C) are not capable of miti- ishing transistor sizes is driven by performance enhancements gating thermal transients during the pulsed load operation, espe- associated with smaller transistors, transistor downscaling is lim- cially when the frequency of the pulses is comparable to the ited by rising leakage currents, and the proportion of static power thermal time constant of the package.
Details
-
File Typepdf
-
Upload Time-
-
Content LanguagesEnglish
-
Upload UserAnonymous/Not logged-in
-
File Pages20 Page
-
File Size-